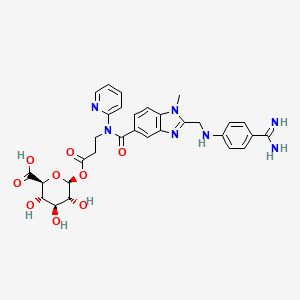
Dabigatran acyl-beta-D-glucuronide
Übersicht
Beschreibung
Dabigatran Acyl-β-D-Glucuronide is a major metabolite of Dabigatran, an orally administered thrombin inhibitor. This compound plays a significant role in the anticoagulant effects of Dabigatran, although it exhibits a weaker inhibitory effect on thrombin generation compared to its parent compound . Dabigatran Acyl-β-D-Glucuronide is formed through the glucuronidation of Dabigatran, a process that enhances its solubility and facilitates its excretion from the body .
Wissenschaftliche Forschungsanwendungen
Dabigatran-Acyl-β-D-Glucuronid hat mehrere Anwendungen in der wissenschaftlichen Forschung, insbesondere in den Bereichen Pharmakologie und Toxikologie. Einige seiner wichtigsten Anwendungen umfassen:
Pharmakokinetik- und Pharmakodynamik-Studien: Forscher verwenden Dabigatran-Acyl-β-D-Glucuronid, um die Pharmakokinetik und Pharmakodynamik von Dabigatran zu untersuchen.
Gerinnungshemmungsforschung: Dabigatran-Acyl-β-D-Glucuronid wird verwendet, um die gerinnungshemmenden Wirkungen von Dabigatran und seinen Metaboliten zu untersuchen.
Studien zum Arzneimittelstoffwechsel: Die Verbindung wird verwendet, um die Stoffwechselwege von Dabigatran und die Rolle von UGT-Enzymen bei seinem Stoffwechsel zu untersuchen.
5. Wirkmechanismus
Dabigatran-Acyl-β-D-Glucuronid übt seine Wirkung aus, indem es Thrombin hemmt, ein Schlüsselenzym in der Gerinnungskaskade. Thrombin katalysiert die Umwandlung von Fibrinogen in Fibrin, was zur Bildung von Blutgerinnseln führt. Durch die Hemmung von Thrombin verhindert Dabigatran-Acyl-β-D-Glucuronid die Bildung von Blutgerinnseln und reduziert das Risiko thromboembolischer Ereignisse . Die Verbindung bindet sowohl an freies als auch an gerinnselgebundenes Thrombin, was sie in verschiedenen klinischen Situationen wirksam macht .
Wirkmechanismus
Target of Action
Dabigatran acyl-beta-D-glucuronide is an active metabolite of the thrombin inhibitor dabigatran . The primary target of this compound is thrombin, a plasma serine protease that plays a central role in coagulation and hemostasis .
Mode of Action
This compound is formed from dabigatran primarily by the UDP-glucuronosyltransferase (UGT) isoform UGT2B15 . It increases activated partial thromboplastin time (aPTT) in isolated human platelet-poor plasma . It exhibits a weaker inhibitory effect than that of dabigatran .
Biochemical Pathways
The glucuronidation of dabigatran, catalyzed by human hepatic and intestinal microsomes, results in the formation of this compound . Three UDP-glucuronosyltransferases (UGTs), namely, UGT1A9, UGT2B7, and UGT2B15, exhibit glucuronidation of dabigatran .
Pharmacokinetics
Dabigatran is administered as the prodrug dabigatran etexilate, which is a substrate of esterases and P-glycoprotein (P-gp). Dabigatran is eliminated via renal excretion but is also a substrate of uridine 5ʹ-diphospho (UDP)-glucuronosyltransferases (UGTs) . Metabolic clearances of dabigatran etexilate and dabigatran were implemented using data on carboxylesterase 1/2 enzymes and UGT subtype 2B15 . In severe renal impairment, the UGT2B15 metabolism and the P-gp transport in the model were reduced to 67% and 65% of the rates in healthy adults .
Result of Action
Both dabigatran and its main metabolite this compound have established anticoagulant effects . They show inhibitory effects on thrombin generation in a dose-dependent manner, but this compound exhibits a weaker inhibitory effect than that of dabigatran .
Action Environment
The action of this compound can be influenced by environmental factors such as the presence of other drugs. For example, drug-drug interaction studies of dabigatran with the P-gp perpetrators rifampin (inducer) and clarithromycin (inhibitor) have been carried out .
Zukünftige Richtungen
Dabigatran has been approved for prophylaxis of thromboembolism in patients undergoing total knee or hip arthroplasty and is being investigated for use in the treatment of venous thromboembolism, prevention of stroke in patients with nonvalvular atrial fibrillation, and treatment of thromboembolic complications, following acute coronary syndromes .
Biochemische Analyse
Biochemical Properties
Dabigatran Acyl-beta-D-glucuronide interacts with several enzymes and proteins in the body. It is formed from dabigatran primarily by the UDP-glucuronosyltransferase (UGT) isoform UGT2B15 . This interaction involves the glucuronidation of the carboxylate moiety of dabigatran .
Cellular Effects
This compound has significant effects on various types of cells and cellular processes. It influences cell function by increasing the activated partial thromboplastin time (aPTT) in isolated human platelet-poor plasma .
Molecular Mechanism
The molecular mechanism of this compound involves its binding interactions with biomolecules and its role in enzyme inhibition or activation. It exerts its effects at the molecular level by inhibiting thrombin, thereby preventing the conversion of fibrinogen to fibrin and impairing the clotting process .
Temporal Effects in Laboratory Settings
In laboratory settings, the effects of this compound change over time. It undergoes non-enzymatic acyl-migration in aqueous solution, resulting in the formation of the 2-O-, 3-O-, and 4-O-acylglucuronides .
Metabolic Pathways
This compound is involved in the metabolic pathway of dabigatran. It is formed from dabigatran primarily by the UDP-glucuronosyltransferase (UGT) isoform UGT2B15 .
Vorbereitungsmethoden
Synthetische Wege und Reaktionsbedingungen: Die Synthese von Dabigatran-Acyl-β-D-Glucuronid beinhaltet die Glucuronidierung von Dabigatran. Diese Reaktion wird durch Uridin-5'-diphospho-glucuronosyltransferase (UGT)-Enzyme katalysiert, insbesondere UGT1A9, UGT2B7 und UGT2B15 . Die Reaktion findet typischerweise in der Leber und im Darm statt, wo diese Enzyme reichlich vorhanden sind. Der Glucuronidierungsprozess beinhaltet die Übertragung einer Glucuronsäure-Einheit an die Carboxylgruppe von Dabigatran, was zur Bildung von Dabigatran-Acyl-β-D-Glucuronid führt .
Industrielle Produktionsmethoden: Die industrielle Produktion von Dabigatran-Acyl-β-D-Glucuronid folgt ähnlichen Prinzipien wie die synthetischen Wege. Das Verfahren beinhaltet die Verwendung menschlicher Leber- und Darm-Mikrosomen, um die Glucuronidierungsreaktion zu katalysieren. Die Reaktionsbedingungen werden optimiert, um eine hohe Ausbeute und Reinheit des Endprodukts zu gewährleisten .
Analyse Chemischer Reaktionen
Arten von Reaktionen: Dabigatran-Acyl-β-D-Glucuronid unterliegt hauptsächlich Hydrolyse- und Transacylierungsreaktionen. Diese Reaktionen sind für seinen Stoffwechsel und seine Ausscheidung entscheidend .
Häufige Reagenzien und Bedingungen:
Hydrolyse: Diese Reaktion beinhaltet die Spaltung der Glucuronsäure-Einheit von Dabigatran-Acyl-β-D-Glucuronid, was zur Bildung von Dabigatran und Glucuronsäure führt.
Transacylierung: Diese Reaktion beinhaltet die Übertragung der Acylgruppe von Dabigatran-Acyl-β-D-Glucuronid auf ein anderes Molekül.
Hauptprodukte, die gebildet werden:
Hydrolyse: Dabigatran und Glucuronsäure.
Transacylierung: Verschiedene acylierte Produkte, abhängig vom Akzeptormolekül.
Vergleich Mit ähnlichen Verbindungen
Dabigatran-Acyl-β-D-Glucuronid ist im Vergleich zu anderen ähnlichen Verbindungen einzigartig aufgrund seines spezifischen Glucuronidierungswegs und seiner Rolle als Hauptmetabolit von Dabigatran. Einige ähnliche Verbindungen umfassen:
Rivaroxaban: Ein weiterer direkter Thrombininhibitor, aber er wird nicht wie Dabigatran glucuronidiert.
Apixaban: Ähnlich wie Rivaroxaban ist es ein direkter Thrombininhibitor mit einem anderen Stoffwechselweg.
Edoxaban: Ein weiterer direkter Thrombininhibitor mit einem unterschiedlichen Stoffwechselprofil.
Diese Verbindungen unterscheiden sich in ihren Stoffwechselwegen, ihrer Pharmakokinetik und Pharmakodynamik, was die Einzigartigkeit von Dabigatran-Acyl-β-D-Glucuronid im Kontext der gerinnungshemmenden Therapie hervorhebt .
Eigenschaften
IUPAC Name |
(2S,3S,4S,5R,6S)-6-[3-[[2-[(4-carbamimidoylanilino)methyl]-1-methylbenzimidazole-5-carbonyl]-pyridin-2-ylamino]propanoyloxy]-3,4,5-trihydroxyoxane-2-carboxylic acid | |
---|---|---|
Source | PubChem | |
URL | https://pubchem.ncbi.nlm.nih.gov | |
Description | Data deposited in or computed by PubChem | |
InChI |
InChI=1S/C31H33N7O9/c1-37-20-10-7-17(14-19(20)36-22(37)15-35-18-8-5-16(6-9-18)28(32)33)29(43)38(21-4-2-3-12-34-21)13-11-23(39)46-31-26(42)24(40)25(41)27(47-31)30(44)45/h2-10,12,14,24-27,31,35,40-42H,11,13,15H2,1H3,(H3,32,33)(H,44,45)/t24-,25-,26+,27-,31+/m0/s1 | |
Source | PubChem | |
URL | https://pubchem.ncbi.nlm.nih.gov | |
Description | Data deposited in or computed by PubChem | |
InChI Key |
CSZFDMHIDSUHPI-KWONYSJQSA-N | |
Source | PubChem | |
URL | https://pubchem.ncbi.nlm.nih.gov | |
Description | Data deposited in or computed by PubChem | |
Canonical SMILES |
CN1C2=C(C=C(C=C2)C(=O)N(CCC(=O)OC3C(C(C(C(O3)C(=O)O)O)O)O)C4=CC=CC=N4)N=C1CNC5=CC=C(C=C5)C(=N)N | |
Source | PubChem | |
URL | https://pubchem.ncbi.nlm.nih.gov | |
Description | Data deposited in or computed by PubChem | |
Isomeric SMILES |
CN1C2=C(C=C(C=C2)C(=O)N(CCC(=O)O[C@H]3[C@@H]([C@H]([C@@H]([C@H](O3)C(=O)O)O)O)O)C4=CC=CC=N4)N=C1CNC5=CC=C(C=C5)C(=N)N | |
Source | PubChem | |
URL | https://pubchem.ncbi.nlm.nih.gov | |
Description | Data deposited in or computed by PubChem | |
Molecular Formula |
C31H33N7O9 | |
Source | PubChem | |
URL | https://pubchem.ncbi.nlm.nih.gov | |
Description | Data deposited in or computed by PubChem | |
DSSTOX Substance ID |
DTXSID60747409 | |
Record name | 1-O-[N-{2-[(4-Carbamimidoylanilino)methyl]-1-methyl-1H-benzimidazole-5-carbonyl}-N-(pyridin-2-yl)-beta-alanyl]-beta-D-glucopyranuronic acid | |
Source | EPA DSSTox | |
URL | https://comptox.epa.gov/dashboard/DTXSID60747409 | |
Description | DSSTox provides a high quality public chemistry resource for supporting improved predictive toxicology. | |
Molecular Weight |
647.6 g/mol | |
Source | PubChem | |
URL | https://pubchem.ncbi.nlm.nih.gov | |
Description | Data deposited in or computed by PubChem | |
CAS No. |
1015167-40-4 | |
Record name | Dabigatran 1-acylglucuronide | |
Source | ChemIDplus | |
URL | https://pubchem.ncbi.nlm.nih.gov/substance/?source=chemidplus&sourceid=1015167404 | |
Description | ChemIDplus is a free, web search system that provides access to the structure and nomenclature authority files used for the identification of chemical substances cited in National Library of Medicine (NLM) databases, including the TOXNET system. | |
Record name | 1-O-[N-{2-[(4-Carbamimidoylanilino)methyl]-1-methyl-1H-benzimidazole-5-carbonyl}-N-(pyridin-2-yl)-beta-alanyl]-beta-D-glucopyranuronic acid | |
Source | EPA DSSTox | |
URL | https://comptox.epa.gov/dashboard/DTXSID60747409 | |
Description | DSSTox provides a high quality public chemistry resource for supporting improved predictive toxicology. | |
Record name | DABIGATRAN 1-ACYLGLUCURONIDE | |
Source | FDA Global Substance Registration System (GSRS) | |
URL | https://gsrs.ncats.nih.gov/ginas/app/beta/substances/U53A719N8O | |
Description | The FDA Global Substance Registration System (GSRS) enables the efficient and accurate exchange of information on what substances are in regulated products. Instead of relying on names, which vary across regulatory domains, countries, and regions, the GSRS knowledge base makes it possible for substances to be defined by standardized, scientific descriptions. | |
Explanation | Unless otherwise noted, the contents of the FDA website (www.fda.gov), both text and graphics, are not copyrighted. They are in the public domain and may be republished, reprinted and otherwise used freely by anyone without the need to obtain permission from FDA. Credit to the U.S. Food and Drug Administration as the source is appreciated but not required. | |
Retrosynthesis Analysis
AI-Powered Synthesis Planning: Our tool employs the Template_relevance Pistachio, Template_relevance Bkms_metabolic, Template_relevance Pistachio_ringbreaker, Template_relevance Reaxys, Template_relevance Reaxys_biocatalysis model, leveraging a vast database of chemical reactions to predict feasible synthetic routes.
One-Step Synthesis Focus: Specifically designed for one-step synthesis, it provides concise and direct routes for your target compounds, streamlining the synthesis process.
Accurate Predictions: Utilizing the extensive PISTACHIO, BKMS_METABOLIC, PISTACHIO_RINGBREAKER, REAXYS, REAXYS_BIOCATALYSIS database, our tool offers high-accuracy predictions, reflecting the latest in chemical research and data.
Strategy Settings
Precursor scoring | Relevance Heuristic |
---|---|
Min. plausibility | 0.01 |
Model | Template_relevance |
Template Set | Pistachio/Bkms_metabolic/Pistachio_ringbreaker/Reaxys/Reaxys_biocatalysis |
Top-N result to add to graph | 6 |
Feasible Synthetic Routes
A: Accurately measuring low concentrations of drugs and their metabolites in complex biological samples like plasma presents a significant analytical challenge. The research article describes a novel three-step stacking capillary electrophoresis (CE) method for the determination of Dabigatran and its active metabolite, Dabigatran acyl-beta-D-glucuronide, in human plasma []. This method, utilizing field-amplified sample injection, sweeping, and analyte focusing by micellar collapse (FASI-sweeping-AFMC), enables the detection of Dabigatran at concentrations as low as 25.2 ng mL−1 in real human plasma samples []. This highlights the method's sensitivity and applicability for clinical assays requiring accurate quantification of these compounds.
Haftungsausschluss und Informationen zu In-Vitro-Forschungsprodukten
Bitte beachten Sie, dass alle Artikel und Produktinformationen, die auf BenchChem präsentiert werden, ausschließlich zu Informationszwecken bestimmt sind. Die auf BenchChem zum Kauf angebotenen Produkte sind speziell für In-vitro-Studien konzipiert, die außerhalb lebender Organismen durchgeführt werden. In-vitro-Studien, abgeleitet von dem lateinischen Begriff "in Glas", beinhalten Experimente, die in kontrollierten Laborumgebungen unter Verwendung von Zellen oder Geweben durchgeführt werden. Es ist wichtig zu beachten, dass diese Produkte nicht als Arzneimittel oder Medikamente eingestuft sind und keine Zulassung der FDA für die Vorbeugung, Behandlung oder Heilung von medizinischen Zuständen, Beschwerden oder Krankheiten erhalten haben. Wir müssen betonen, dass jede Form der körperlichen Einführung dieser Produkte in Menschen oder Tiere gesetzlich strikt untersagt ist. Es ist unerlässlich, sich an diese Richtlinien zu halten, um die Einhaltung rechtlicher und ethischer Standards in Forschung und Experiment zu gewährleisten.