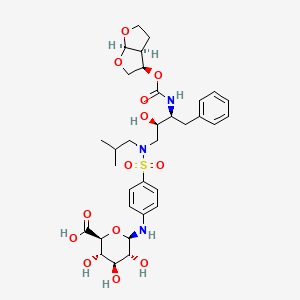
DARUNAVIR N-BETA-D-GLUCURONIDE
Übersicht
Beschreibung
Darunavir N-beta-D-glucuronide: is a metabolite of darunavir, an inhibitor of the human immunodeficiency virus (HIV) protease. Darunavir is used in combination with other antiretroviral agents for the treatment of HIV-1 infection. The glucuronide form is a result of the glucuronidation process, which is a common phase II metabolic pathway to eliminate small molecule drugs from the body .
Vorbereitungsmethoden
Synthetic Routes and Reaction Conditions: The preparation of darunavir N-beta-D-glucuronide involves the glucuronidation of darunavir. This process can be achieved through enzymatic reactions using liver microsomes or recombinant enzymes. The reaction typically involves the use of uridine 5’-diphosphoglucuronic acid (UDPGA) as the glucuronic acid donor .
Industrial Production Methods: Industrial production of this compound can involve large-scale enzymatic reactions using bioreactors. The process includes the isolation and purification of the glucuronide product using techniques such as liquid chromatography and high-resolution mass spectrometry .
Analyse Chemischer Reaktionen
Types of Reactions: Darunavir N-beta-D-glucuronide primarily undergoes hydrolysis and conjugation reactions. The glucuronide moiety can be cleaved under acidic or basic conditions, leading to the release of the parent compound, darunavir .
Common Reagents and Conditions:
Hydrolysis: Acidic or basic conditions using hydrochloric acid or sodium hydroxide.
Conjugation: UDPGA in the presence of liver microsomes or recombinant enzymes.
Major Products Formed:
Hydrolysis: Darunavir
Conjugation: This compound
Wissenschaftliche Forschungsanwendungen
Pharmacokinetics and Metabolism
Darunavir undergoes extensive metabolism in the liver, primarily through cytochrome P450 enzymes, particularly CYP3A4. The formation of Darunavir N-Beta-D-Glucuronide occurs via glucuronidation processes mediated by uridine diphosphate-glucuronosyltransferases (UGTs) . Understanding the pharmacokinetics of this metabolite is crucial for optimizing dosing regimens and predicting drug interactions.
Table 1: Pharmacokinetic Parameters of Darunavir and Its Glucuronides
Parameter | Darunavir | This compound |
---|---|---|
Bioavailability | 37% - 82% | Not directly measured |
Cmax (ng/mL) | 2282 - 3578 | Not specified |
Half-life (hours) | Approximately 15-20 | Not specified |
Protein Binding | ~95% | Not specified |
Metabolism | Hepatic (CYP3A4) | UGT-mediated |
Therapeutic Efficacy in HIV Treatment
Darunavir is primarily indicated for the treatment of HIV-1 infection. Studies have shown that darunavir/ritonavir combination therapy is effective in both treatment-naïve and treatment-experienced patients. The presence of its glucuronide metabolite may influence therapeutic outcomes by modulating drug levels and activity in different tissues, including the central nervous system (CNS) .
Case Study: Efficacy in Adolescents
A clinical trial assessed once-daily darunavir/ritonavir (800/100 mg) in treatment-naïve adolescents over 48 weeks. Results indicated significant viral load reductions and increases in CD4 cell counts, demonstrating the effectiveness of this regimen . The role of this compound in this context remains an area for further investigation, particularly regarding its impact on drug bioavailability and safety profiles.
Potential Repurposing in Oncology
Recent studies have explored the potential of darunavir and its metabolites, including this compound, in cancer therapy. Research suggests that darunavir may exhibit anti-tumor properties by inhibiting specific cancer cell pathways. For instance, its ability to synergize with other chemotherapeutic agents like paclitaxel has been observed .
Table 2: Potential Anti-Cancer Mechanisms
Mechanism | Description |
---|---|
Inhibition of Tumor Growth | Demonstrated in preclinical models |
Synergistic Effects | Enhanced efficacy when combined with paclitaxel |
Targeting Specific Pathways | Potential inhibition of angiogenesis |
Safety Profile and Drug Interactions
The safety profile of darunavir is well-documented, with adverse effects generally being mild to moderate. However, the formation of glucuronides may alter the pharmacodynamics and safety profile of darunavir, necessitating careful monitoring for potential drug interactions .
Case Study: CNS Concentration Analysis
A study measuring darunavir concentrations in cerebrospinal fluid (CSF) found that unbound darunavir levels exceeded inhibitory concentrations for HIV, suggesting effective CNS penetration . The implications for this compound's role in this context warrant further exploration to understand its impact on HIV management within the CNS.
Wirkmechanismus
Darunavir N-beta-D-glucuronide itself does not have a direct mechanism of action as it is a metabolite. darunavir, the parent compound, inhibits the HIV-1 protease enzyme, preventing the cleavage of viral polyproteins and thereby inhibiting viral replication . The glucuronidation of darunavir facilitates its excretion from the body, contributing to the overall pharmacokinetic profile of the drug .
Vergleich Mit ähnlichen Verbindungen
- Darunavir O-glucuronide
- Darunavir acyl-glucuronide
- Raltegravir beta-D-glucuronide
- Dolutegravir beta-D-glucuronide
Comparison: Darunavir N-beta-D-glucuronide is unique in its structure and metabolic pathway compared to other glucuronide metabolites. While darunavir O-glucuronide and acyl-glucuronide are also metabolites of darunavir, they differ in the site of glucuronidation. Raltegravir and dolutegravir glucuronides are metabolites of different antiretroviral drugs and have distinct pharmacokinetic and pharmacodynamic profiles .
Biologische Aktivität
Darunavir (DRV) is a widely used protease inhibitor in the treatment of HIV-1 infection. Its biological activity is significantly influenced by its metabolic pathways, particularly through the formation of metabolites such as Darunavir N-Beta-D-Glucuronide (DRV-N-Gluc). This article explores the biological activity of DRV-N-Gluc, including its pharmacokinetics, interactions with cytochrome P450 enzymes, and implications for therapeutic efficacy.
Overview of Darunavir and Its Metabolism
Darunavir is characterized by its ability to inhibit the HIV-1 protease, which is essential for viral replication. The drug exhibits strong binding affinity to the active site of the protease, with an effective concentration (EC50) ranging from 1 to 5 nM against various HIV strains . Upon administration, DRV undergoes extensive hepatic metabolism primarily via cytochrome P450 enzymes, notably CYP3A4 .
Formation of this compound
The metabolic pathway leading to the formation of DRV-N-Gluc involves glucuronidation, a phase II metabolic reaction mediated by uridine 5'-diphosphate-glucuronosyltransferases (UGTs). This process typically results in the conjugation of glucuronic acid to drugs, enhancing their solubility and facilitating excretion .
Pharmacokinetics
The pharmacokinetic profile of DRV is marked by high protein binding (approximately 95%), predominantly to alpha-1-acid glycoprotein and albumin . The formation of DRV-N-Gluc significantly impacts its pharmacokinetics:
- Half-life : The terminal elimination half-life of DRV is approximately 41 hours .
- Bioavailability : The bioavailability of DRV is influenced by food intake and co-administration with other antiretroviral agents like ritonavir or cobicistat .
Table 1: Pharmacokinetic Parameters of Darunavir
Parameter | Value |
---|---|
Protein Binding | ~95% |
AUC (24h) | 93,026–124,698 ng·h/mL |
Cmax | 2282–3578 ng/mL |
Terminal Half-Life | ~41 hours |
Interaction with Cytochrome P450 Enzymes
Research indicates that DRV-N-Gluc exhibits minimal inhibitory effects on CYP enzymes compared to its parent compound. While glucuronides generally have reduced enzymatic activity, some studies suggest that certain glucuronides can inhibit specific CYP isoforms weakly .
Table 2: Inhibition Profiles of Darunavir and Its Glucuronides on CYP Enzymes
Compound | CYP2C19 Inhibition | CYP3A4 Inhibition |
---|---|---|
Darunavir | Moderate | Strong |
Darunavir N-Beta-D-Gluc | Weak | Minimal |
Clinical Implications and Case Studies
The clinical significance of DRV-N-Gluc lies in its potential impact on drug interactions and therapeutic efficacy. Case studies have shown that patients receiving DRV treatment may experience variations in drug efficacy based on individual metabolic profiles, particularly concerning UGT polymorphisms that influence glucuronidation rates.
Case Study Example
A study involving treatment-experienced patients demonstrated that those with higher levels of DRV-N-Gluc had lower viral loads compared to those with minimal glucuronide formation. This suggests a potential role for monitoring glucuronide levels as a biomarker for therapeutic response in HIV treatment regimens .
Eigenschaften
IUPAC Name |
(2S,3S,4S,5R,6R)-6-[4-[[(2R,3S)-3-[[(3aS,4R,6aR)-2,3,3a,4,5,6a-hexahydrofuro[2,3-b]furan-4-yl]oxycarbonylamino]-2-hydroxy-4-phenylbutyl]-(2-methylpropyl)sulfamoyl]anilino]-3,4,5-trihydroxyoxane-2-carboxylic acid | |
---|---|---|
Source | PubChem | |
URL | https://pubchem.ncbi.nlm.nih.gov | |
Description | Data deposited in or computed by PubChem | |
InChI |
InChI=1S/C33H45N3O13S/c1-18(2)15-36(50(44,45)21-10-8-20(9-11-21)34-30-28(40)26(38)27(39)29(49-30)31(41)42)16-24(37)23(14-19-6-4-3-5-7-19)35-33(43)48-25-17-47-32-22(25)12-13-46-32/h3-11,18,22-30,32,34,37-40H,12-17H2,1-2H3,(H,35,43)(H,41,42)/t22-,23-,24+,25-,26-,27-,28+,29-,30+,32+/m0/s1 | |
Source | PubChem | |
URL | https://pubchem.ncbi.nlm.nih.gov | |
Description | Data deposited in or computed by PubChem | |
InChI Key |
XXXHCLBMCZVFGB-VMMQOENWSA-N | |
Source | PubChem | |
URL | https://pubchem.ncbi.nlm.nih.gov | |
Description | Data deposited in or computed by PubChem | |
Canonical SMILES |
CC(C)CN(CC(C(CC1=CC=CC=C1)NC(=O)OC2COC3C2CCO3)O)S(=O)(=O)C4=CC=C(C=C4)NC5C(C(C(C(O5)C(=O)O)O)O)O | |
Source | PubChem | |
URL | https://pubchem.ncbi.nlm.nih.gov | |
Description | Data deposited in or computed by PubChem | |
Isomeric SMILES |
CC(C)CN(C[C@H]([C@H](CC1=CC=CC=C1)NC(=O)O[C@H]2CO[C@@H]3[C@H]2CCO3)O)S(=O)(=O)C4=CC=C(C=C4)N[C@H]5[C@@H]([C@H]([C@@H]([C@H](O5)C(=O)O)O)O)O | |
Source | PubChem | |
URL | https://pubchem.ncbi.nlm.nih.gov | |
Description | Data deposited in or computed by PubChem | |
Molecular Formula |
C33H45N3O13S | |
Source | PubChem | |
URL | https://pubchem.ncbi.nlm.nih.gov | |
Description | Data deposited in or computed by PubChem | |
DSSTOX Substance ID |
DTXSID90747417 | |
Record name | N-{4-[{(2R,3S)-3-[({[(3R,3aS,6aR)-Hexahydrofuro[2,3-b]furan-3-yl]oxy}carbonyl)amino]-2-hydroxy-4-phenylbutyl}(2-methylpropyl)sulfamoyl]phenyl}-beta-D-glucopyranuronosylamine | |
Source | EPA DSSTox | |
URL | https://comptox.epa.gov/dashboard/DTXSID90747417 | |
Description | DSSTox provides a high quality public chemistry resource for supporting improved predictive toxicology. | |
Molecular Weight |
723.8 g/mol | |
Source | PubChem | |
URL | https://pubchem.ncbi.nlm.nih.gov | |
Description | Data deposited in or computed by PubChem | |
CAS No. |
1159613-25-8 | |
Record name | Darunavir metabolite M18 | |
Source | ChemIDplus | |
URL | https://pubchem.ncbi.nlm.nih.gov/substance/?source=chemidplus&sourceid=1159613258 | |
Description | ChemIDplus is a free, web search system that provides access to the structure and nomenclature authority files used for the identification of chemical substances cited in National Library of Medicine (NLM) databases, including the TOXNET system. | |
Record name | N-{4-[{(2R,3S)-3-[({[(3R,3aS,6aR)-Hexahydrofuro[2,3-b]furan-3-yl]oxy}carbonyl)amino]-2-hydroxy-4-phenylbutyl}(2-methylpropyl)sulfamoyl]phenyl}-beta-D-glucopyranuronosylamine | |
Source | EPA DSSTox | |
URL | https://comptox.epa.gov/dashboard/DTXSID90747417 | |
Description | DSSTox provides a high quality public chemistry resource for supporting improved predictive toxicology. | |
Record name | 1-DEOXY-1-((4-((((2R,3S)-3-(((((3R,3AS,6AR)-HEXAHYDROFURO(2,3-B)FURAN-3-YL)OXY)CARBONYL)AMINO)-2-HYDROXY-4-PHENYLBUTYL)(2-METHYLPROPYL)AMINO)SULFONYL)PHENYL)AMINO)-.BETA.-D-GLUCOPYRANURONIC ACID | |
Source | FDA Global Substance Registration System (GSRS) | |
URL | https://gsrs.ncats.nih.gov/ginas/app/beta/substances/KU641CZY53 | |
Description | The FDA Global Substance Registration System (GSRS) enables the efficient and accurate exchange of information on what substances are in regulated products. Instead of relying on names, which vary across regulatory domains, countries, and regions, the GSRS knowledge base makes it possible for substances to be defined by standardized, scientific descriptions. | |
Explanation | Unless otherwise noted, the contents of the FDA website (www.fda.gov), both text and graphics, are not copyrighted. They are in the public domain and may be republished, reprinted and otherwise used freely by anyone without the need to obtain permission from FDA. Credit to the U.S. Food and Drug Administration as the source is appreciated but not required. | |
Retrosynthesis Analysis
AI-Powered Synthesis Planning: Our tool employs the Template_relevance Pistachio, Template_relevance Bkms_metabolic, Template_relevance Pistachio_ringbreaker, Template_relevance Reaxys, Template_relevance Reaxys_biocatalysis model, leveraging a vast database of chemical reactions to predict feasible synthetic routes.
One-Step Synthesis Focus: Specifically designed for one-step synthesis, it provides concise and direct routes for your target compounds, streamlining the synthesis process.
Accurate Predictions: Utilizing the extensive PISTACHIO, BKMS_METABOLIC, PISTACHIO_RINGBREAKER, REAXYS, REAXYS_BIOCATALYSIS database, our tool offers high-accuracy predictions, reflecting the latest in chemical research and data.
Strategy Settings
Precursor scoring | Relevance Heuristic |
---|---|
Min. plausibility | 0.01 |
Model | Template_relevance |
Template Set | Pistachio/Bkms_metabolic/Pistachio_ringbreaker/Reaxys/Reaxys_biocatalysis |
Top-N result to add to graph | 6 |
Feasible Synthetic Routes
Haftungsausschluss und Informationen zu In-Vitro-Forschungsprodukten
Bitte beachten Sie, dass alle Artikel und Produktinformationen, die auf BenchChem präsentiert werden, ausschließlich zu Informationszwecken bestimmt sind. Die auf BenchChem zum Kauf angebotenen Produkte sind speziell für In-vitro-Studien konzipiert, die außerhalb lebender Organismen durchgeführt werden. In-vitro-Studien, abgeleitet von dem lateinischen Begriff "in Glas", beinhalten Experimente, die in kontrollierten Laborumgebungen unter Verwendung von Zellen oder Geweben durchgeführt werden. Es ist wichtig zu beachten, dass diese Produkte nicht als Arzneimittel oder Medikamente eingestuft sind und keine Zulassung der FDA für die Vorbeugung, Behandlung oder Heilung von medizinischen Zuständen, Beschwerden oder Krankheiten erhalten haben. Wir müssen betonen, dass jede Form der körperlichen Einführung dieser Produkte in Menschen oder Tiere gesetzlich strikt untersagt ist. Es ist unerlässlich, sich an diese Richtlinien zu halten, um die Einhaltung rechtlicher und ethischer Standards in Forschung und Experiment zu gewährleisten.