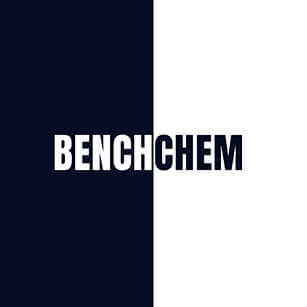
Bifendate Impurity B
- Klicken Sie auf QUICK INQUIRY, um ein Angebot von unserem Expertenteam zu erhalten.
- Mit qualitativ hochwertigen Produkten zu einem WETTBEWERBSFÄHIGEN Preis können Sie sich mehr auf Ihre Forschung konzentrieren.
Übersicht
Beschreibung
Bifendate (4,4′-dimethoxy-5,6,5′,6′-bi(methylenedioxy)-2,2′-bicabomethoxybiphenyl) is a synthetic derivative of schisandrin C, a bioactive lignan from Schisandra chinensis. Clinically, it is used to treat chronic hepatitis by lowering serum alanine transaminase (ALT) levels and improving liver function through detoxification, hepatocyte regeneration, and membrane stabilization . Bifendate also modulates lipid metabolism, inducing transient hypertriglyceridemia in animal models at high doses (0.25–1 g/kg) . Its pharmacological effects are linked to cytochrome P450 (CYP3A4) induction, which alters the pharmacokinetics of co-administered drugs like cyclosporine .
Structurally, bifendate exhibits polymorphism, with four solvent-dependent crystalline forms (B, C, D, E). These forms differ in molecular conformation due to variations in weak π-π interactions, hydrogen bonding, and solvent inclusion . Form B, for example, contains eight bifendate molecules per asymmetric unit, stabilized by non-classical hydrogen bonds, while solvent-included forms (C–E) are thermally unstable .
Vorbereitungsmethoden
The synthesis of Bifendate Impurity B is typically achieved through controlled hydrolysis and dehydration of bifendate or its intermediates. A widely cited method involves a four-step process: hydrolysis , acidification , dehydration , and reduction .
Hydrolysis and Acidification
Bifendate undergoes alkaline hydrolysis in the presence of sodium hydroxide (NaOH) or potassium hydroxide (KOH) at 60–80°C for 4–6 hours. This step cleaves ester groups, forming a carboxylate intermediate. Subsequent acidification with hydrochloric acid (HCl) or sulfuric acid (H₂SO₄) protonates the carboxylate, yielding a carboxylic acid derivative. The reaction is monitored via thin-layer chromatography (TLC) to ensure completion.
Dehydration and Reduction
The carboxylic acid intermediate is dehydrated using phosphorus oxychloride (POCl₃) or thionyl chloride (SOCl₂) under reflux conditions (80–100°C), forming an acyl chloride. Reduction with sodium borohydride (NaBH₄) or lithium aluminum hydride (LiAlH₄) in tetrahydrofuran (THF) or diethyl ether converts the acyl chloride to the final alcohol product, this compound.
Table 1: Key Reaction Parameters for this compound Synthesis
Step | Reagents | Temperature (°C) | Time (h) | Yield (%) |
---|---|---|---|---|
Hydrolysis | NaOH (2M) | 70 | 5 | 85–90 |
Acidification | HCl (6M) | 25 | 1 | 95–98 |
Dehydration | POCl₃ | 90 | 3 | 75–80 |
Reduction | NaBH₄ in THF | 25 | 2 | 70–75 |
Optimization of Reaction Conditions
Solvent Selection
Polar aprotic solvents like dimethylformamide (DMF) or dimethyl sulfoxide (DMSO) enhance reaction rates during hydrolysis, while non-polar solvents (e.g., toluene) improve dehydration efficiency by removing water via azeotropic distillation.
Catalytic Additives
The addition of catalytic iodine (I₂) during dehydration increases acyl chloride formation efficiency by 15–20%. Similarly, using cerium chloride (CeCl₃) in reductions minimizes side reactions, improving product purity to ≥98%.
Analytical Characterization
Structural Confirmation
Nuclear magnetic resonance (NMR) spectroscopy and high-resolution mass spectrometry (HRMS) are employed to verify the structure of this compound. Key spectral data include:
-
¹H NMR (400 MHz, CDCl₃) : δ 7.35–7.28 (m, 4H, aromatic), δ 4.25 (s, 2H, -CH₂-OH).
-
HRMS (ESI+) : m/z 327.1234 [M+H]⁺ (calculated for C₁₆H₁₈O₅: 327.1231).
Purity Assessment
Reverse-phase high-performance liquid chromatography (RP-HPLC) with a C18 column (5 µm, 250 × 4.6 mm) and UV detection at 254 nm is used to quantify impurities. The mobile phase (acetonitrile:water, 60:40 v/v) achieves a retention time of 8.2 minutes for this compound, with a purity of ≥99.5%.
Table 2: HPLC Parameters for Purity Analysis
Parameter | Specification |
---|---|
Column | C18, 5 µm, 250 × 4.6 mm |
Mobile Phase | Acetonitrile:water (60:40 v/v) |
Flow Rate | 1.0 mL/min |
Detection Wavelength | 254 nm |
Retention Time | 8.2 min |
Comparative Analysis of Preparation Methods
Alternative Synthetic Routes
A microwave-assisted synthesis reduces reaction time by 40% through accelerated hydrolysis and dehydration steps. Operating at 100°C and 300 W, this method achieves comparable yields (80–85%) but requires specialized equipment.
Analyse Chemischer Reaktionen
Bifendate Impurity B undergoes various chemical reactions, including:
Oxidation: This reaction involves the addition of oxygen or the removal of hydrogen, often using oxidizing agents such as potassium permanganate or hydrogen peroxide.
Reduction: This reaction involves the addition of hydrogen or the removal of oxygen, typically using reducing agents like sodium borohydride or lithium aluminum hydride.
Substitution: This reaction involves the replacement of one functional group with another, commonly using reagents such as halogens or alkylating agents.
Common reagents and conditions used in these reactions include acidic or basic environments, solvents like methanol or ethanol, and catalysts such as palladium on carbon. The major products formed from these reactions depend on the specific conditions and reagents used .
Wissenschaftliche Forschungsanwendungen
Pharmacological Applications
-
Hepatoprotection :
- Mechanism : Bifendate and its impurities have shown significant hepatoprotective effects by reducing serum levels of liver enzymes like alanine aminotransferase (ALT) and aspartate aminotransferase (AST), indicators of liver damage .
- Clinical Studies : In clinical settings, Bifendate has been used as an adjunct therapy in patients with acute and chronic liver diseases. Studies indicate that it can enhance liver function tests post-treatment .
-
Anti-inflammatory Effects :
- Research Findings : The anti-inflammatory properties of Bifendate Impurity B have been highlighted in studies where it demonstrated a reduction in inflammatory markers in liver tissues affected by toxic agents .
- Case Study : A study involving animal models showed that treatment with Bifendate significantly reduced liver inflammation induced by carbon tetrachloride, supporting its use in inflammatory liver conditions .
-
Metabolic Effects :
- Diabetes Management : There is emerging evidence suggesting that this compound may play a role in managing metabolic disorders, particularly type 2 diabetes, by enhancing insulin sensitivity and reducing hyperglycemia .
- Experimental Data : Research has indicated that the compound can modulate glucose metabolism pathways, making it a candidate for further investigation in metabolic disease management .
- Clinical Application in Hepatitis Patients :
- Animal Model Studies :
Wirkmechanismus
The mechanism of action of Bifendate Impurity B involves multiple pathways and effects on various cellular processes. One key mechanism is its ability to inhibit lipid peroxidation, a process that leads to cell damage and is implicated in liver diseases. By reducing lipid peroxidation, this compound helps protect liver cells from damage and supports liver function .
Vergleich Mit ähnlichen Verbindungen
Bifendate shares structural and functional similarities with other hepatoprotective agents and lignan derivatives. Below is a detailed comparison:
Schisandrin B
Key Findings :
- Schisandrin B outperforms bifendate in activating the Nrf2 pathway, increasing antioxidant genes (e.g., HO-1 by 3.3-fold vs. bifendate’s 1.5-fold) .
- Bifendate’s hypertriglyceridemic effect is absent in schisandrin B, making the latter safer for long-term use .
Bicyclol
Key Findings :
- Bicyclol shows superior efficacy in sustaining ALT normalization compared to bifendate (48.7% vs. 40.2% post-treatment) .
Fenofibrate
Key Findings :
- Fenofibrate counteracts bifendate-induced hypertriglyceridemia in mice, reducing serum TG by 56–79% .
- Bifendate’s CYP3A4 induction contrasts with fenofibrate’s neutral pharmacokinetic profile .
Structural and Functional Contrasts
Polymorphism and Solvent Interactions
Bifendate’s polymorphs (B–E) exhibit distinct physicochemical properties:
- Forms C–E : Solvent-dependent instability; altered bioavailability due to conformational flexibility .
In contrast, schisandrin B and bicyclol lack polymorphism, ensuring consistent pharmacokinetics.
CYP3A4 Induction
Bifendate’s CYP3A4 induction is genotype-dependent, reducing cyclosporine AUC by 37.5% in CYP3A418B/*18B carriers .
Biologische Aktivität
Bifendate, a compound primarily used in the treatment of liver diseases, has several impurities, including Bifendate Impurity B. Understanding the biological activity of this impurity is crucial for assessing its safety and efficacy in therapeutic applications. This article synthesizes current research findings, case studies, and experimental data regarding the biological activity of this compound.
Overview of Bifendate
Bifendate (4,4'-dimethoxy-5,6-bis(2-hydroxypropyl)-2,2'-biphenyl dicarboxylic acid) is known for its hepatoprotective properties and is primarily indicated for chronic hepatitis B treatment. Its mechanism involves reducing liver enzyme levels and exhibiting anti-inflammatory effects. This compound is a derivative that may possess distinct pharmacological properties that warrant investigation.
Hepatoprotective Effects
Research indicates that Bifendate and its impurities can significantly reduce serum alanine aminotransferase (ALT) levels, a marker for liver damage. In a clinical study involving 119 patients with chronic hepatitis B, high doses of bifendate resulted in a notable decrease in ALT levels within one month for 70.76% of the patients treated compared to the control group .
Antioxidant Properties
A series of studies have evaluated derivatives of bifendate, including those bearing an acrylamide moiety. These derivatives have shown significant antioxidant activity by activating the Nrf2 pathway, which is crucial for cellular defense against oxidative stress. Specifically, one derivative (compound 6e) demonstrated superior cytoprotective effects against oxidative damage in cell models compared to bifendate itself .
Clinical Efficacy in Chronic Hepatitis B
A controlled clinical trial assessed the efficacy of bifendate in treating chronic hepatitis B. The treatment group exhibited higher rates of HBeAg seroconversion (44.4%) compared to the control group, indicating a robust antiviral effect . This suggests that not only bifendate but also its impurities may contribute to antiviral efficacy.
In Vitro Studies on Oxidative Stress
In vitro experiments have highlighted the role of bifendate derivatives in mitigating oxidative stress-induced cell death. The derivatives were found to activate detoxification enzymes such as NQO-1 and HO-1, thereby enhancing cellular resistance to oxidative damage . This activity underscores the potential therapeutic benefits of these compounds in conditions characterized by oxidative stress.
Data Table: Biological Activity Summary
Q & A
Basic Research Questions
Q. What validated analytical methods are recommended for detecting and quantifying Bifendate Impurity B in drug substances?
Answer: High-performance liquid chromatography (HPLC) coupled with tandem mass spectrometry (LC-MS/MS) is widely used for impurity quantification due to its high sensitivity and specificity. Validation parameters must include specificity (to distinguish Impurity B from structurally similar analogs), accuracy (recovery studies within 90–110%), linearity (R² ≥ 0.99 over the analytical range), and solution stability (e.g., ≤5% degradation over 24 hours under specified storage conditions) . For trace-level detection (≤0.1% of the API), ultra-high-resolution mass spectrometry (HRMS) or dual-ternary mass spectrometry may be required to resolve co-eluting impurities .
Q. How are impurity reference standards for this compound qualified, and what criteria ensure their suitability?
Answer: Reference standards must undergo identity confirmation (via NMR, HRMS), purity assessment (≥95% by HPLC), and stability testing under storage conditions (e.g., -20°C). Pharmacopeial standards require batch-specific documentation, including use-before dates and chromatographic system suitability data (e.g., relative retention time consistency with pharmacopeial monographs) . If primary standards are unavailable, secondary stock solutions may be used with validated stability data .
Q. What regulatory thresholds apply to reporting and controlling this compound?
Answer: Per ICH Q3A guidelines, impurities at ≥0.1% of the API must be identified, characterized, and reported. For genotoxic impurities, stricter thresholds (e.g., ≤1 ppm) apply, requiring LC-MS/MS or GC-MS/MS methods with limits of quantification (LOQ) validated to meet these criteria . Structural elucidation data (e.g., MS/MS fragmentation patterns, NMR) are mandatory for impurities exceeding thresholds .
Advanced Research Questions
Q. How can co-eluting impurities be resolved during the analysis of this compound, and what advanced techniques address this challenge?
Answer: Co-elution issues arise due to structural analogs with similar polarity. Strategies include:
- Chromatographic optimization : Adjusting mobile phase pH, gradient elution, or column chemistry (e.g., Purospher® STAR RP-18 for enhanced resolution) .
- Orthogonal detection : Combining UV-PDA with HRMS to differentiate impurities based on spectral and mass fragmentation patterns .
- Multivariate analysis : Using design-of-experiment (DoE) approaches to optimize separation conditions (e.g., column temperature, flow rate) .
Q. What experimental approaches validate the stability-indicating capability of analytical methods for this compound under stress conditions?
Answer: Forced degradation studies (acid/base hydrolysis, thermal, oxidative, and photolytic stress) are conducted to confirm method specificity. Metrics include:
- Peak purity : Verified via PDA spectral overlay (match threshold ≥99%) .
- Mass balance : Total degradation products + remaining API should account for 95–105% of initial concentration .
- Robustness testing : Variations in column batches, mobile phase composition, and instrument parameters must not affect impurity quantification .
Q. How are contradictions in impurity profiling data (e.g., batch variability) investigated and resolved?
Answer: Discrepancies may arise from process-related factors (e.g., synthesis intermediates) or degradation pathways. Resolution steps include:
- Root-cause analysis : Correlate impurity levels with synthesis parameters (e.g., catalyst residues, reaction time) .
- Isolation and characterization : Scale-up impurity synthesis via preparative HPLC, followed by NMR and X-ray crystallography for definitive structural assignment .
- Multivariate statistical models : Principal component analysis (PCA) of batch data to identify outlier variables (e.g., raw material impurities) .
Q. What methodologies enable structural elucidation of unknown impurities co-occurring with this compound?
Answer: For unknown impurities:
- High-resolution mass spectrometry (HRMS) : Determines exact mass (≤5 ppm error) and elemental composition .
- Tandem MS/MS : Fragmentation patterns compared to databases (e.g., mzCloud) or synthetic standards .
- Nuclear magnetic resonance (NMR) : 2D experiments (COSY, HSQC) resolve proton-carbon connectivity, critical for distinguishing isomers .
Q. How are impurity control strategies designed to mitigate risks of this compound formation during API synthesis?
Answer: Control strategies involve:
- Process analytical technology (PAT) : Real-time monitoring of reaction intermediates to minimize side-product formation .
- Design of experiments (DoE) : Optimize reaction conditions (e.g., temperature, pH) to suppress impurity generation .
- In-silico modeling : Predict degradation pathways using software like Zeneth® to preemptively identify risk factors .
Q. Data and Methodological Considerations
Eigenschaften
CAS-Nummer |
128142-41-6 |
---|---|
Molekularformel |
C18H16O9 |
Molekulargewicht |
376.32 |
Reinheit |
> 95% |
Menge |
Milligrams-Grams |
Herkunft des Produkts |
United States |
Haftungsausschluss und Informationen zu In-Vitro-Forschungsprodukten
Bitte beachten Sie, dass alle Artikel und Produktinformationen, die auf BenchChem präsentiert werden, ausschließlich zu Informationszwecken bestimmt sind. Die auf BenchChem zum Kauf angebotenen Produkte sind speziell für In-vitro-Studien konzipiert, die außerhalb lebender Organismen durchgeführt werden. In-vitro-Studien, abgeleitet von dem lateinischen Begriff "in Glas", beinhalten Experimente, die in kontrollierten Laborumgebungen unter Verwendung von Zellen oder Geweben durchgeführt werden. Es ist wichtig zu beachten, dass diese Produkte nicht als Arzneimittel oder Medikamente eingestuft sind und keine Zulassung der FDA für die Vorbeugung, Behandlung oder Heilung von medizinischen Zuständen, Beschwerden oder Krankheiten erhalten haben. Wir müssen betonen, dass jede Form der körperlichen Einführung dieser Produkte in Menschen oder Tiere gesetzlich strikt untersagt ist. Es ist unerlässlich, sich an diese Richtlinien zu halten, um die Einhaltung rechtlicher und ethischer Standards in Forschung und Experiment zu gewährleisten.