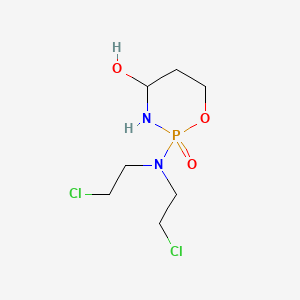
4-Hydroxycyclophosphamide
- Klicken Sie auf QUICK INQUIRY, um ein Angebot von unserem Expertenteam zu erhalten.
- Mit qualitativ hochwertigen Produkten zu einem WETTBEWERBSFÄHIGEN Preis können Sie sich mehr auf Ihre Forschung konzentrieren.
Übersicht
Beschreibung
4-Hydroxycyclophosphamide is a significant compound in the class of oxazaphosphorine compounds. It is the main active metabolite of cyclophosphamide and mafosfamide, which are widely used in chemotherapy . This compound is crucial due to its role in the metabolic pathway of cyclophosphamide, a prodrug used to treat various cancers and autoimmune disorders .
Vorbereitungsmethoden
Synthetic Routes and Reaction Conditions: The synthesis of 4-Hydroxycyclophosphamide involves the hydroxylation of cyclophosphamide. This process can be catalyzed by enzymes such as cytochrome P450 in the liver . An alternative method involves the use of unspecific peroxygenases from fungi like Marasmius rotula, which can hydroxylate cyclophosphamide efficiently .
Industrial Production Methods: Industrial production of this compound typically involves the enzymatic hydroxylation of cyclophosphamide using cytochrome P450 enzymes. This method is preferred due to its high specificity and efficiency .
Analyse Chemischer Reaktionen
Arten von Reaktionen: 4-Hydroxycyclophosphamid unterliegt verschiedenen Arten von Reaktionen, darunter:
Oxidation: Es kann zu 4-Ketocyclophosphamid oxidiert werden.
Hydrolyse: Es kann zu Phosphoramid-Senfgas und Acrolein hydrolysiert werden, die beide zytotoxisch sind.
Häufige Reagenzien und Bedingungen:
Oxidation: Wasserstoffperoxid wird häufig in Gegenwart von Peroxygenasen verwendet.
Hydrolyse: Diese Reaktion findet unter physiologischen Bedingungen innerhalb von Zellen statt.
Hauptprodukte:
Phosphoramid-Senfgas: Eine zytotoxische Verbindung, die DNA alkyliert.
Acrolein: Eine toxische Verbindung, die Zellschäden verursachen kann.
Wissenschaftliche Forschungsanwendungen
4-Hydroxycyclophosphamid hat zahlreiche Anwendungen in der wissenschaftlichen Forschung:
Chemie: Es wird verwendet, um die Stoffwechselwege von Cyclophosphamid und seinen Derivaten zu untersuchen.
5. Wirkmechanismus
4-Hydroxycyclophosphamid entfaltet seine Wirkung durch verschiedene Mechanismen:
DNA-Alkylierung: Es wird in Phosphoramid-Senfgas umgewandelt, das DNA alkyliert, was zum Zelltod führt.
Apoptoseinduktion: Die Verbindung induziert Apoptose durch die Bildung von Hydroxypropanal, was die zytotoxischen Wirkungen verstärkt.
Stoffwechselwege: Es wird zu Aldophosphamid metabolisiert, das weiter zu zytotoxischen Metaboliten umgewandelt wird.
Wirkmechanismus
4-Hydroxycyclophosphamide exerts its effects through several mechanisms:
DNA Alkylation: It is converted to phosphoramide mustard, which alkylates DNA, leading to cell death.
Apoptosis Induction: The compound induces apoptosis through the formation of hydroxypropanal, which amplifies the cytotoxic effects.
Metabolic Pathways: It is metabolized to aldophosphamide, which is further converted to cytotoxic metabolites.
Vergleich Mit ähnlichen Verbindungen
4-Hydroxycyclophosphamid ist aufgrund seiner spezifischen Rolle als aktiver Metabolit von Cyclophosphamid einzigartig. Ähnliche Verbindungen umfassen:
Cyclophosphamid: Die Stammverbindung, die zu 4-Hydroxycyclophosphamid metabolisiert wird.
Mafosfamid: Eine weitere Oxazaphosphorin-Verbindung, die auf ähnliche Weise metabolisiert wird.
Phosphoramid-Senfgas: Ein direkter zytotoxischer Metabolit, der aus 4-Hydroxycyclophosphamid gebildet wird.
Acrolein: Ein toxisches Nebenprodukt des 4-Hydroxycyclophosphamid-Stoffwechsels.
4-Hydroxycyclophosphamid zeichnet sich durch seine spezifische Rolle bei der metabolischen Aktivierung von Cyclophosphamid aus, was es zu einer wichtigen Verbindung in der Chemotherapieforschung und -anwendung macht.
Biologische Aktivität
4-Hydroxycyclophosphamide (4-OH-CPA) is a significant metabolite of cyclophosphamide, a widely used alkylating agent in cancer chemotherapy. This compound exhibits both therapeutic and toxic biological activities, primarily through its metabolism and interactions within biological systems. Understanding the biological activity of 4-OH-CPA is crucial for optimizing cancer treatment regimens and minimizing adverse effects.
Cyclophosphamide is converted into this compound via cytochrome P450 enzymes, particularly CYP2B6, CYP2C9, and CYP3A4. This conversion is essential for its antitumor efficacy as 4-OH-CPA can spontaneously decompose into phosphoramide mustard, the active alkylating metabolite responsible for cytotoxicity against cancer cells .
Key Metabolic Pathways:
- Conversion to Active Metabolites:
- Cyclophosphamide → this compound → Phosphoramide Mustard (active) + Acrolein (toxic by-product)
- Tautomerization:
Antitumor Effects
This compound exhibits significant antitumor activity, which is closely correlated with the area under the curve (AUC) of its concentration in plasma. Studies indicate that maximizing exposure (AUC) through continuous infusion rather than bolus injection enhances therapeutic efficacy . The cytotoxic effects of phosphoramide mustard are reported to be 4 to 10 times greater than those of 4-OH-CPA itself .
Toxicity
While 4-OH-CPA is less cytotoxic at physiological pH, it can lead to myelosuppression and other toxicities due to the formation of acrolein, which is responsible for hemorrhagic cystitis and bladder toxicity . The balance between therapeutic efficacy and toxicity is a critical consideration in clinical settings.
Pharmacokinetics
The pharmacokinetics of this compound has been studied in various populations, including cancer patients and individuals with glomerulonephritis. Research has shown that genetic polymorphisms in enzymes like CYP2B6 can significantly alter the metabolism of cyclophosphamide and its active metabolite .
Pharmacokinetic Parameters:
- Cmax (Maximum Concentration): Correlates with myelosuppression effects.
- AUC (Area Under Curve): Correlates with antitumor activity.
Case Studies
-
Cancer Treatment Efficacy:
A study involving patients with various cancers demonstrated that higher AUC values for 4-OH-CPA were associated with better treatment outcomes. Continuous infusion protocols showed improved efficacy compared to traditional dosing methods . -
Impact on Glomerulonephritis:
In patients with lupus nephritis, altered pharmacokinetics were observed for both cyclophosphamide and 4-OH-CPA, indicating that renal function significantly impacts drug disposition and efficacy .
Summary Table of Biological Activities
Eigenschaften
CAS-Nummer |
61903-30-8 |
---|---|
Molekularformel |
C7H15Cl2N2O3P |
Molekulargewicht |
277.08 g/mol |
IUPAC-Name |
(2R,4S)-2-[bis(2-chloroethyl)amino]-2-oxo-1,3,2λ5-oxazaphosphinan-4-ol |
InChI |
InChI=1S/C7H15Cl2N2O3P/c8-2-4-11(5-3-9)15(13)10-7(12)1-6-14-15/h7,12H,1-6H2,(H,10,13)/t7-,15+/m0/s1 |
InChI-Schlüssel |
RANONBLIHMVXAJ-NZFNHWASSA-N |
SMILES |
C1COP(=O)(NC1O)N(CCCl)CCCl |
Isomerische SMILES |
C1CO[P@](=O)(N[C@H]1O)N(CCCl)CCCl |
Kanonische SMILES |
C1COP(=O)(NC1O)N(CCCl)CCCl |
Aussehen |
Low Melting Off-White to Pale Yellow Solid |
Reinheit |
> 95% |
Menge |
Milligrams-Grams |
Synonyme |
(2R,4S)-rel-2-[Bis(2-chloroethyl)amino]tetrahydro-2H-1,3,2-oxazaphosphorin-4-ol 2-Oxide |
Herkunft des Produkts |
United States |
Haftungsausschluss und Informationen zu In-Vitro-Forschungsprodukten
Bitte beachten Sie, dass alle Artikel und Produktinformationen, die auf BenchChem präsentiert werden, ausschließlich zu Informationszwecken bestimmt sind. Die auf BenchChem zum Kauf angebotenen Produkte sind speziell für In-vitro-Studien konzipiert, die außerhalb lebender Organismen durchgeführt werden. In-vitro-Studien, abgeleitet von dem lateinischen Begriff "in Glas", beinhalten Experimente, die in kontrollierten Laborumgebungen unter Verwendung von Zellen oder Geweben durchgeführt werden. Es ist wichtig zu beachten, dass diese Produkte nicht als Arzneimittel oder Medikamente eingestuft sind und keine Zulassung der FDA für die Vorbeugung, Behandlung oder Heilung von medizinischen Zuständen, Beschwerden oder Krankheiten erhalten haben. Wir müssen betonen, dass jede Form der körperlichen Einführung dieser Produkte in Menschen oder Tiere gesetzlich strikt untersagt ist. Es ist unerlässlich, sich an diese Richtlinien zu halten, um die Einhaltung rechtlicher und ethischer Standards in Forschung und Experiment zu gewährleisten.