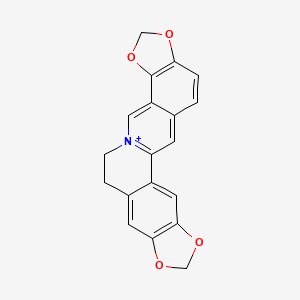
Coptisine
Beschreibung
Molecular Characteristics and Chemical Properties
Coptisine possesses the molecular formula C19H14NO4+ with a molecular weight of 320.3 grams per mole. The compound exists as a quaternary ammonium alkaloid, which contributes significantly to its unique chemical behavior and biological activities. The systematic chemical name for this compound is 6,7-dihydrobis(1,3)benzodioxolo(5,6-a:4',5'-g)quinolizinium, reflecting its complex polycyclic structure.
The compound exhibits distinctive structural features that include multiple methylenedioxy groups and a quinolizinium core, which are characteristic of the isoquinoline alkaloid family. This structural configuration contributes to the compound's stability and its ability to interact with various biological targets. The Chemical Abstracts Service registry number for this compound is 3486-66-6, providing a unique identifier for this compound in chemical databases.
Physical and Chemical Properties
This compound demonstrates several notable physical characteristics that are important for both its isolation and potential applications. The compound appears as yellowish needles when crystallized from alcohol, with a melting point of 218 degrees Celsius. In its various salt forms, this compound exhibits different physical properties that are relevant for different applications and research purposes.
The chloride salt of this compound, known as this compound chloride, appears as orange prisms that do not melt at temperatures up to 300 degrees Celsius. The iodide form presents as yellow needles that decompose above 280 degrees Celsius, while the sulfate form manifests as yellow crystals. These variations in physical properties among different salt forms provide researchers with options for different experimental conditions and applications.
Solubility characteristics of this compound vary significantly depending on the solvent and salt form. The compound is very sparingly soluble in water and sparingly soluble in alcohol, but shows solubility in alkaline solutions. The sulfate form is notably insoluble in both water and alcohol, which enables its separation from related alkaloids such as berberine and worenine.
Eigenschaften
IUPAC Name |
5,7,17,19-tetraoxa-13-azoniahexacyclo[11.11.0.02,10.04,8.015,23.016,20]tetracosa-1(13),2,4(8),9,14,16(20),21,23-octaene | |
---|---|---|
Source | PubChem | |
URL | https://pubchem.ncbi.nlm.nih.gov | |
Description | Data deposited in or computed by PubChem | |
InChI |
InChI=1S/C19H14NO4/c1-2-16-19(24-10-21-16)14-8-20-4-3-12-6-17-18(23-9-22-17)7-13(12)15(20)5-11(1)14/h1-2,5-8H,3-4,9-10H2/q+1 | |
Source | PubChem | |
URL | https://pubchem.ncbi.nlm.nih.gov | |
Description | Data deposited in or computed by PubChem | |
InChI Key |
XYHOBCMEDLZUMP-UHFFFAOYSA-N | |
Source | PubChem | |
URL | https://pubchem.ncbi.nlm.nih.gov | |
Description | Data deposited in or computed by PubChem | |
Canonical SMILES |
C1C[N+]2=C(C=C3C=CC4=C(C3=C2)OCO4)C5=CC6=C(C=C51)OCO6 | |
Source | PubChem | |
URL | https://pubchem.ncbi.nlm.nih.gov | |
Description | Data deposited in or computed by PubChem | |
Molecular Formula |
C19H14NO4+ | |
Source | PubChem | |
URL | https://pubchem.ncbi.nlm.nih.gov | |
Description | Data deposited in or computed by PubChem | |
Related CAS |
6020-18-4 (chloride) | |
Record name | Coptisine | |
Source | ChemIDplus | |
URL | https://pubchem.ncbi.nlm.nih.gov/substance/?source=chemidplus&sourceid=0003486666 | |
Description | ChemIDplus is a free, web search system that provides access to the structure and nomenclature authority files used for the identification of chemical substances cited in National Library of Medicine (NLM) databases, including the TOXNET system. | |
DSSTOX Substance ID |
DTXSID10188404 | |
Record name | Coptisine | |
Source | EPA DSSTox | |
URL | https://comptox.epa.gov/dashboard/DTXSID10188404 | |
Description | DSSTox provides a high quality public chemistry resource for supporting improved predictive toxicology. | |
Molecular Weight |
320.3 g/mol | |
Source | PubChem | |
URL | https://pubchem.ncbi.nlm.nih.gov | |
Description | Data deposited in or computed by PubChem | |
CAS No. |
3486-66-6 | |
Record name | Coptisine | |
Source | CAS Common Chemistry | |
URL | https://commonchemistry.cas.org/detail?cas_rn=3486-66-6 | |
Description | CAS Common Chemistry is an open community resource for accessing chemical information. Nearly 500,000 chemical substances from CAS REGISTRY cover areas of community interest, including common and frequently regulated chemicals, and those relevant to high school and undergraduate chemistry classes. This chemical information, curated by our expert scientists, is provided in alignment with our mission as a division of the American Chemical Society. | |
Explanation | The data from CAS Common Chemistry is provided under a CC-BY-NC 4.0 license, unless otherwise stated. | |
Record name | Coptisine | |
Source | ChemIDplus | |
URL | https://pubchem.ncbi.nlm.nih.gov/substance/?source=chemidplus&sourceid=0003486666 | |
Description | ChemIDplus is a free, web search system that provides access to the structure and nomenclature authority files used for the identification of chemical substances cited in National Library of Medicine (NLM) databases, including the TOXNET system. | |
Record name | Coptisine | |
Source | EPA DSSTox | |
URL | https://comptox.epa.gov/dashboard/DTXSID10188404 | |
Description | DSSTox provides a high quality public chemistry resource for supporting improved predictive toxicology. | |
Record name | COPTISINE | |
Source | FDA Global Substance Registration System (GSRS) | |
URL | https://gsrs.ncats.nih.gov/ginas/app/beta/substances/0GCL71VN14 | |
Description | The FDA Global Substance Registration System (GSRS) enables the efficient and accurate exchange of information on what substances are in regulated products. Instead of relying on names, which vary across regulatory domains, countries, and regions, the GSRS knowledge base makes it possible for substances to be defined by standardized, scientific descriptions. | |
Explanation | Unless otherwise noted, the contents of the FDA website (www.fda.gov), both text and graphics, are not copyrighted. They are in the public domain and may be republished, reprinted and otherwise used freely by anyone without the need to obtain permission from FDA. Credit to the U.S. Food and Drug Administration as the source is appreciated but not required. | |
Record name | Coptisine | |
Source | Hazardous Substances Data Bank (HSDB) | |
URL | https://pubchem.ncbi.nlm.nih.gov/source/hsdb/8323 | |
Description | The Hazardous Substances Data Bank (HSDB) is a toxicology database that focuses on the toxicology of potentially hazardous chemicals. It provides information on human exposure, industrial hygiene, emergency handling procedures, environmental fate, regulatory requirements, nanomaterials, and related areas. The information in HSDB has been assessed by a Scientific Review Panel. | |
Vorbereitungsmethoden
Acid-Based Extraction from Raw Materials
The most widely used industrial method involves sulfuric acid extraction from Coptis chinensis rhizomes. Key steps include:
-
Raw Material Pretreatment : Crushed rhizomes (20–100 mesh) are immersed in 0.1–5% sulfuric acid (3–15× volume) at 20–100°C for 1–48 hours.
-
Multi-Stage Extraction : Four sequential extractions optimize yield:
-
Selective Precipitation : Filtrates are treated with 0.1–10% H₂SO₄ and 1–20% sulfates (e.g., Na₂SO₄) at 0–30°C for 1–100 hours, achieving 50–90% this compound purity.
Critical Parameters :
Factor | Optimal Range | Impact on Yield |
---|---|---|
Sulfuric Acid Conc. | 0.05–1% (w/v) | ↑ Solubility |
Extraction Temp | 80–100°C | ↑ Kinetics |
Solid:Liquid Ratio | 1:5–1:15 (w/v) | ↑ Diffusion |
This method achieves 7.8 mg/g this compound yield but requires careful pH control to avoid alkaloid degradation.
Modern Chromatographic Approaches
High-Speed Countercurrent Chromatography (HSCCC)
A biphasic solvent system (n-hexane:ethyl acetate:methanol:water = 2:5:2:5 v/v) enables isolation of this compound at 88.5% purity. Operational parameters:
-
Flow Rate: 2 mL/min (initial), 5 mL/min (final)
-
Rotation Speed: 850→650 rpm gradient
-
Run Time: 5 hours
Performance Comparison :
Alkaloid | Purity (%) | Recovery (%) |
---|---|---|
This compound | 88.5 | 72.3 |
Berberine | 95.2 | 85.1 |
Palmatine | 91.7 | 78.9 |
While effective for small-scale purification, HSCCC shows limited scalability for industrial production.
Chemical Synthesis Pathways
Hydrogenation-Demethylation Route
Starting from mixed Coptis alkaloids (berberine/palmatine):
-
Hydrogenation :
-
Demethylation :
Limitations : Multi-step synthesis introduces byproducts (e.g., de-methylated analogs), requiring rigorous purification.
Solvent Optimization Studies
Organic Solvent Screening
Comparative analysis of 16 solvents revealed:
Solvent | Conc. (%) | This compound Yield (mg/g) |
---|---|---|
Methanol | 75 | 7.8 ± 0.3 |
Ethanol | 75 | 7.6 ± 0.2 |
Acetone | 50 | 5.1 ± 0.4 |
0.4% H₂SO₄ | - | 6.9 ± 0.5 |
Methanol showed superior extraction efficiency (p < 0.05) due to optimal polarity matching this compound's logP ≈ 1.2.
Deep Eutectic Solvents (DESs)
Novel DES formulations improved yields by 18–22% versus traditional solvents:
DES Component | Molar Ratio | This compound Yield (mg/g) |
---|---|---|
ChCl:LA | 1:2 | 9.2 ± 0.4 |
ChCl:CA | 1:1 | 8.7 ± 0.3 |
ChCl:Gly | 1:1 | 8.1 ± 0.2 |
Choline chloride-lactic acid (ChCl:LA) DES achieved 9.2 mg/g yield through enhanced hydrogen bonding with alkaloid N-oxides.
Industrial-Scale Process Design
Economic Analysis of Methods
Method | Capacity (kg/day) | Cost ($/kg) | Purity (%) |
---|---|---|---|
Acid Extraction | 500–1000 | 120–150 | 50–90 |
HSCCC | 5–10 | 950–1200 | 85–95 |
Synthetic | 1–2 | 4200–5000 | 98+ |
Acid extraction remains dominant for bulk production, while HSCCC serves niche pharmaceutical applications requiring >90% purity.
Analyse Chemischer Reaktionen
Anti-inflammatory Effects
- Coptisine inhibits the production of pro-inflammatory mediators. Studies show that this compound can inhibit the production of nitric oxide (NO) by suppressing the protein and mRNA expressions of inducible nitric oxide synthase (iNOS) in lipopolysaccharide (LPS)-stimulated RAW 264.7 macrophages .
- It also reduces the levels of pro-inflammatory cytokines such as interleukin-1beta (IL-1beta) and interleukin-6 (IL-6) by suppressing the expression of cytokine mRNA .
- This compound suppresses the degradation of inhibitor of nuclear factor kappaBalpha (IkappaBalpha) and the phosphorylation of extracellular signal-regulated kinase (ERK), c-Jun NH2-terminal kinase (JNK), p38 mitogen-activated protein kinase (MAPK), and phosphoinositide 3-kinase/Akt (PI3K/Akt) .
- In LPS-stimulated rats, this compound inhibited plasma TNF-α production in a linear manner, which led to a reduction of iNOS and NO .
Anti-tumor Effects
- This compound induces apoptosis in tumor cells. It affects apoptosis-associated targets like ROS, Bcl-2/-XL, Bid, Bax, cytochrome c, Apaf-a, AIF, XIAP, caspase-3, and caspase-9 .
- It can induce cell cycle arrest in the G1 phase and decrease the expression of CDK4, CDK2, cyclin E, and cyclin C, which are key genes in the G1/S phase .
- This compound promotes DNA damage and triggers apoptosis by up-regulating ROS, caspase-3/-9, Bax/Bcl-2, and PARP cleavage .
- This compound also displayed antiproliferative action against vascular smooth muscle cells (VSMCs) by blocking the cell cycle at G(1) and G(2)/M phases . The G(1) block involves a decrease in cyclin D1 protein due to accelerated proteolysis via the proteasome-dependent pathway .
Antibacterial Effects
- This compound has antibacterial activity against Pasteurella multocida, with a minimum inhibitory concentration (MIC) of 0.125 mg/mL .
- It inhibits the activity of succinate dehydrogenase (SDH) and lactate dehydrogenase (LDH), which are involved in the respiratory system of P. multocida .
- This compound inhibits soluble protein synthesis in P. multocida .
Induction of Apoptosis in Hepatocellular Carcinoma Cells
- This compound induces apoptosis in Hep3B hepatocellular carcinoma cells through the activation of the ROS-mediated JNK signaling pathway .
- This compound enhances DNA damage, activates both extrinsic and intrinsic apoptosis pathways, and induces mitochondrial dysfunction in Hep3B cells .
- It increases intracellular and mitochondrial ROS production and regulates ROS-dependent apoptosis in Hep3B cells .
This compound's effects on cell cycle arrest and apoptosis involve modulation of various proteins and enzymes . For instance, in HCT-116 cells, this compound induces G0/G1 cell cycle arrest, decreasing Cyclin D1 and Cyclin E levels . The compound also triggers caspase-dependent apoptotic pathways .
Wissenschaftliche Forschungsanwendungen
Koptisin hat eine breite Palette von Anwendungen in der wissenschaftlichen Forschung:
Medizin: Koptisin hat sich bei der Behandlung verschiedener Krankheiten, darunter Krebs, Diabetes und Herz-Kreislauf-Erkrankungen, als vielversprechend erwiesen.
5. Wirkmechanismus
Koptisin entfaltet seine Wirkungen über mehrere molekulare Ziele und Signalwege:
Antimikrobielle Aktivität: Koptisin stört die Zellwandpermeabilität von Bakterien, hemmt respiratorische Enzyme und stört die DNA-Synthese.
Entzündungshemmende Aktivität: Koptisin moduliert Signalwege wie NF-κB und MAPK und reduziert die Produktion von pro-inflammatorischen Zytokinen.
Antikrebsaktivität: Koptisin induziert Apoptose in Krebszellen, indem es Kaspasen-Signalwege aktiviert und die Zellproliferation hemmt.
Wirkmechanismus
Coptisine is often compared with other protoberberine alkaloids such as berberine, palmatine, and jatrorrhizine. While all these compounds share similar chemical structures, this compound is unique in its higher efficacy against certain cancer cell lines and its distinct molecular targets .
Vergleich Mit ähnlichen Verbindungen
Structural Analogues: Berberine and Palmatine
Coptisine, berberine, and palmatine are co-occurring isoquinoline alkaloids in Coptis species, differing in methoxy and methylenedioxy substituents (Table 1). These structural variations influence their lipophilicity and target specificity:
- This compound : Lacks a methyl group at position 9, enhancing its interaction with urease and calcium channels .
- Berberine : A methyl group at position 9 increases its affinity for antimicrobial targets .
- Reduced derivatives : Tetrahydrothis compound and dihydrothis compound exhibit weaker transannular interactions (density <0.11 vs. 0.11 in this compound), reducing bioactivity .
Table 1. Structural and Functional Comparison of this compound with Analogues
*Approximate values based on chromatographic data .
Pharmacological Activity Comparison
Anti-Inflammatory Effects
This compound uniquely inhibits Rho/ROCK and NF-κB pathways, reducing TNF-α and IL-17 in lupus and psoriasis models . In contrast, berberine primarily modulates AMPK and gut microbiota for metabolic inflammation .
Anticancer Mechanisms
This compound selectively induces apoptosis in liver cancer cells via 67LR/cGMP, sparing normal hepatocytes . This mechanism is distinct from berberine’s broad-spectrum cell cycle arrest and palmatine’s weaker pro-apoptotic effects.
Urease Inhibition
This compound (IC₅₀ ~2.5 µM) is 10–100× more potent than BA and NaF, which competitively bind nickel ions in urease . However, BA/NaF partially protect Helicobacter pylori urease (HPU) from this compound, suggesting this compound targets non-nickel sites like thiol groups .
Table 2. Urease Inhibition Efficacy
Pharmacokinetic and Mechanistic Insights
This compound’s anti-inflammatory PK-PD profile follows a linear model, where plasma concentration directly correlates with TNF-α inhibition . Its rapid action (0.5 h post-LPS) contrasts with slower-acting alkaloids like berberine, which require prolonged exposure for AMPK activation .
Biologische Aktivität
Coptisine, a prominent alkaloid derived from the roots of Coptis chinensis, has garnered significant attention in pharmacological research due to its diverse biological activities. This article provides a comprehensive overview of the biological activity of this compound, including its antibacterial, anti-inflammatory, and anticancer properties, supported by relevant case studies and research findings.
Chemical Structure and Properties
This compound is classified as a protoberberine alkaloid, characterized by its unique chemical structure which contributes to its biological activities. The molecular formula of this compound is C_20H_19NO_4, with a molecular weight of 339.37 g/mol. Its structure allows it to interact with various biological targets, making it a subject of interest in medicinal chemistry.
Antibacterial Activity
This compound exhibits potent antibacterial properties, particularly against Gram-negative bacteria such as Pasteurella multocida. Recent studies have demonstrated that this compound has a minimum inhibitory concentration (MIC) of 0.125 mg/mL , indicating strong antibacterial efficacy .
The antibacterial mechanism involves several pathways:
- Cell Wall Disruption : this compound alters cell wall permeability, leading to cell lysis.
- Inhibition of Respiratory Enzymes : It significantly reduces the activity of succinate dehydrogenase (SDH) and lactate dehydrogenase (LDH), crucial for bacterial respiration. For instance, SDH activity decreased from 31.53 ± 1.27 U/mg protein in untreated cells to 5.80 ± 0.74 U/mg protein in treated cells .
- Protein Synthesis Inhibition : this compound treatment resulted in a significant reduction in soluble protein synthesis within bacterial cells .
Anti-inflammatory Activity
This compound has shown promising anti-inflammatory effects in various animal models. In a study involving xylene-induced ear edema and carrageenan-induced paw edema models, this compound significantly reduced inflammation markers such as interleukin-1β (IL-1β) and tumor necrosis factor (TNF-α) .
Signaling Pathways
The anti-inflammatory action is mediated through inhibition of key signaling pathways:
- NF-κB Pathway : this compound inhibited the translocation of NF-κB components, thereby reducing inflammatory responses.
- MAPK Pathway : It also blocked the phosphorylation of p38 and JNK MAPK pathways, contributing to its anti-inflammatory effects .
Anticancer Activity
Emerging research indicates that this compound possesses anticancer properties. It has been shown to induce apoptosis in various cancer cell lines through mechanisms involving caspase activation and modulation of cell cycle regulators.
Case Studies
- Breast Cancer Cells : this compound treatment led to significant apoptosis in MCF-7 breast cancer cells, with increased caspase-3 activity observed.
- Liver Cancer Models : In hepatocellular carcinoma models, this compound demonstrated cytotoxic effects by inhibiting cell proliferation and inducing cell cycle arrest.
Comparative Biological Activities
A comparative analysis of this compound with other alkaloids from Coptis chinensis reveals its superior efficacy against Helicobacter pylori, highlighting its potential as a therapeutic agent for gastric disorders .
Biological Activity | This compound | Other Alkaloids |
---|---|---|
Antibacterial | Strong | Moderate |
Anti-inflammatory | High | Variable |
Anticancer | Significant | Low |
Q & A
Q. Q1. What validated methods are recommended for isolating and quantifying coptisine from plant matrices?
this compound isolation typically involves solvent extraction (e.g., ethanol, methanol) combined with chromatographic techniques like HPLC or UPLC. For quantification, standard calibration curves using purified this compound (≥98% purity) are essential. Method validation should include recovery rates (aim for >90%) and precision tests (RSD <5%) . Recent studies suggest ultrasound-assisted extraction improves yield by 15–20% compared to traditional Soxhlet methods, though solvent polarity and pH must be optimized for specific plant species .
Q. Q2. How do researchers address discrepancies in this compound’s reported bioactivity across in vitro and in vivo studies?
Contradictions often arise due to differences in bioavailability, metabolic conversion, or experimental models. For example, this compound’s anti-inflammatory effects in vitro (IC₅₀ ~10 µM) may not translate directly to in vivo models due to poor intestinal absorption. Methodological solutions include:
Q. Q3. What are the standard assays for evaluating this compound’s antioxidant activity, and how should interference from co-extracted compounds be minimized?
Common assays include DPPH radical scavenging, FRAP, and ORAC. To mitigate interference:
- Pre-treat extracts with solid-phase extraction (SPE) to remove phenolic acids or flavonoids.
- Include negative controls (e.g., solvent-only samples) and cross-validate with LC-MS to confirm this compound’s contribution to observed activity .
Advanced Research Questions
Q. Q4. How can researchers design experiments to elucidate this compound’s molecular targets in complex pathways, such as AMPK/mTOR signaling?
A structured approach involves:
Target Prediction : Use cheminformatics tools (e.g., SwissTargetPrediction) to identify putative binding proteins.
Validation : Apply siRNA knockdown or CRISPR-Cas9 gene editing in relevant cell lines to confirm pathway involvement.
Functional Assays : Measure downstream markers (e.g., p-AMPK, LC3-II for autophagy) under this compound treatment.
Dose-Response Analysis : Establish EC₅₀ values and compare with known pathway modulators .
Q. Q5. What strategies resolve contradictory data on this compound’s hepatoprotective vs. hepatotoxic effects?
Contradictions may stem from dose-dependent effects or model-specific responses. Solutions include:
- Toxicogenomics : Profile gene expression changes (e.g., CYP450 enzymes) in primary hepatocytes exposed to varying this compound concentrations (1–100 µM).
- Metabolomic Profiling : Identify toxic metabolites (e.g., oxidized derivatives) using LC-HRMS.
- Species Sensitivity Analysis : Compare results across human, rat, and zebrafish models to assess translational relevance .
Q. Q6. How can researchers optimize this compound’s synergistic effects with other alkaloids (e.g., berberine) in polyherbal formulations?
Use factorial design experiments to evaluate interactions:
- Combination Index (CI) : Calculate via the Chou-Talalay method (CI <1 indicates synergy).
- Mechanistic Studies : Assess shared targets (e.g., NF-κB inhibition) using dual-luciferase reporter assays.
- Pharmacokinetic Synergy : Monitor co-administration effects on absorption and half-life in rodent models .
Q. Q7. What analytical frameworks are suitable for evaluating this compound’s epigenetic modifications (e.g., DNA methylation, histone acetylation)?
Recommended methodologies:
- Methylated DNA Immunoprecipitation (MeDIP-seq) : Identify genome-wide methylation changes.
- ChIP-seq : Map histone acetylation at promoter regions of target genes (e.g., tumor suppressors).
- Integrative Analysis : Combine omics data (methylome, transcriptome) to establish causal relationships .
Methodological Considerations
Q. Q8. How should researchers control for batch-to-batch variability in this compound sourcing?
Q. Q9. What statistical approaches are robust for analyzing this compound’s dose-response relationships in heterogeneous cell populations?
Q. Q10. How can computational modeling (e.g., molecular docking, QSAR) enhance this compound’s structure-activity relationship (SAR) studies?
- Docking Simulations : Use AutoDock Vina to predict binding affinities for targets like acetylcholinesterase.
- QSAR Models : Train on datasets of this compound analogs to predict bioavailability or toxicity .
Featured Recommendations
Most viewed | ||
---|---|---|
Most popular with customers |
Haftungsausschluss und Informationen zu In-Vitro-Forschungsprodukten
Bitte beachten Sie, dass alle Artikel und Produktinformationen, die auf BenchChem präsentiert werden, ausschließlich zu Informationszwecken bestimmt sind. Die auf BenchChem zum Kauf angebotenen Produkte sind speziell für In-vitro-Studien konzipiert, die außerhalb lebender Organismen durchgeführt werden. In-vitro-Studien, abgeleitet von dem lateinischen Begriff "in Glas", beinhalten Experimente, die in kontrollierten Laborumgebungen unter Verwendung von Zellen oder Geweben durchgeführt werden. Es ist wichtig zu beachten, dass diese Produkte nicht als Arzneimittel oder Medikamente eingestuft sind und keine Zulassung der FDA für die Vorbeugung, Behandlung oder Heilung von medizinischen Zuständen, Beschwerden oder Krankheiten erhalten haben. Wir müssen betonen, dass jede Form der körperlichen Einführung dieser Produkte in Menschen oder Tiere gesetzlich strikt untersagt ist. Es ist unerlässlich, sich an diese Richtlinien zu halten, um die Einhaltung rechtlicher und ethischer Standards in Forschung und Experiment zu gewährleisten.