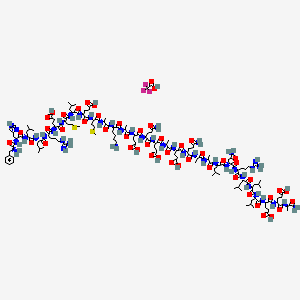
a-Helical Corticotropin Releasing Factor (12-41)
- Klicken Sie auf QUICK INQUIRY, um ein Angebot von unserem Expertenteam zu erhalten.
- Mit qualitativ hochwertigen Produkten zu einem WETTBEWERBSFÄHIGEN Preis können Sie sich mehr auf Ihre Forschung konzentrieren.
Übersicht
Beschreibung
a-Helical Corticotropin Releasing Factor (12-41) is a synthetic peptide that mimics the structure and function of corticotropin-releasing factor, a hormone produced in the hypothalamus. This compound is composed of 30 amino acids and adopts an alpha-helical conformation. It is primarily used in scientific research to study the regulation of adrenocorticotropic hormone secretion and the body’s response to stress .
Vorbereitungsmethoden
Synthetic Routes and Reaction Conditions
The synthesis of a-Helical Corticotropin Releasing Factor (12-41) typically involves solid-phase peptide synthesis (SPPS). This method allows for the sequential addition of amino acids to a growing peptide chain anchored to a solid resin. The process includes:
Coupling: Each amino acid is activated and coupled to the resin-bound peptide chain.
Deprotection: Temporary protecting groups on the amino acids are removed to allow for the next coupling step.
Cleavage: The completed peptide is cleaved from the resin and purified.
Common reagents used in SPPS include N,N’-diisopropylcarbodiimide (DIC) for activation and trifluoroacetic acid (TFA) for deprotection .
Industrial Production Methods
While specific industrial production methods for a-Helical Corticotropin Releasing Factor (12-41) are not widely documented, large-scale peptide synthesis generally follows similar principles as laboratory-scale SPPS but with optimizations for efficiency and yield. Automated peptide synthesizers and high-performance liquid chromatography (HPLC) are often employed for purification .
Analyse Chemischer Reaktionen
Types of Reactions
a-Helical Corticotropin Releasing Factor (12-41) primarily undergoes peptide bond formation and cleavage reactions during its synthesis. It can also participate in:
Oxidation: Oxidative conditions can affect the peptide’s methionine residues.
Reduction: Disulfide bonds, if present, can be reduced to free thiols.
Substitution: Amino acid residues can be substituted to create analogs with different properties.
Common Reagents and Conditions
Oxidation: Hydrogen peroxide or performic acid.
Reduction: Dithiothreitol (DTT) or tris(2-carboxyethyl)phosphine (TCEP).
Substitution: Various amino acid derivatives and coupling reagents.
Major Products
The major products formed from these reactions are typically modified peptides with altered biological activity or stability. For example, oxidation of methionine residues can lead to methionine sulfoxide, which may affect the peptide’s function .
Wissenschaftliche Forschungsanwendungen
a-Helical Corticotropin Releasing Factor (12-41) has a wide range of applications in scientific research:
Chemistry: Used as a model peptide to study peptide synthesis and modification techniques.
Biology: Investigates the role of corticotropin-releasing factor in stress response and hormone regulation.
Medicine: Explores potential therapeutic applications in stress-related disorders and endocrine diseases.
Industry: Utilized in the development of peptide-based drugs and diagnostic tools.
Wirkmechanismus
a-Helical Corticotropin Releasing Factor (12-41) exerts its effects by binding to corticotropin-releasing factor receptors (CRF receptors) on target cells. This binding inhibits the stimulatory effect of endogenous corticotropin-releasing factor on adrenocorticotropic hormone secretion. The primary molecular targets are the CRF receptors, which are G-protein-coupled receptors involved in the stress response pathway .
Vergleich Mit ähnlichen Verbindungen
Similar Compounds
Corticotropin-Releasing Factor (CRF): The natural hormone that stimulates adrenocorticotropic hormone secretion.
Urocortins: Peptides related to corticotropin-releasing factor with similar biological activities.
Sauvagine: A peptide from amphibians with corticotropin-releasing factor-like activity.
Uniqueness
a-Helical Corticotropin Releasing Factor (12-41) is unique in its ability to inhibit the action of endogenous corticotropin-releasing factor, making it a valuable tool for studying the regulation of the stress response and potential therapeutic applications .
Eigenschaften
IUPAC Name |
(4S)-5-[[(2S)-5-amino-1-[[(2S)-1-[[(2S)-1-[[(2S)-1-[[(2S)-5-amino-1-[[(2S)-1-[[(2S)-1-[[(2S)-1-[[(2S)-4-amino-1-[[(2S)-1-[[(2S)-1-[[(2S)-1-[[(2S)-1-[[(2S)-1-[[(2S)-1-[[(2S)-1-amino-1-oxopropan-2-yl]amino]-4-carboxy-1-oxobutan-2-yl]amino]-4-carboxy-1-oxobutan-2-yl]amino]-4-methyl-1-oxopentan-2-yl]amino]-4-methyl-1-oxopentan-2-yl]amino]-4-methyl-1-oxopentan-2-yl]amino]-5-carbamimidamido-1-oxopentan-2-yl]amino]-1,4-dioxobutan-2-yl]amino]-4-methyl-1-oxopentan-2-yl]amino]-1-oxopropan-2-yl]amino]-1-oxopropan-2-yl]amino]-1,5-dioxopentan-2-yl]amino]-4-carboxy-1-oxobutan-2-yl]amino]-1-oxopropan-2-yl]amino]-4-carboxy-1-oxobutan-2-yl]amino]-1,5-dioxopentan-2-yl]amino]-4-[[(2S)-2-[[(2S)-6-amino-2-[[(2S)-2-[[(2S)-2-[[(2S)-2-[[(2S)-2-[[(2S)-2-[[(2S)-2-[[(2S)-2-[[(2S)-2-[[(2S)-2-[[(2S)-2-[[(2S)-2-amino-3-phenylpropanoyl]amino]-3-(1H-imidazol-4-yl)propanoyl]amino]-4-methylpentanoyl]amino]-4-methylpentanoyl]amino]-5-carbamimidamidopentanoyl]amino]-4-carboxybutanoyl]amino]-4-methylsulfanylbutanoyl]amino]-4-methylpentanoyl]amino]-4-carboxybutanoyl]amino]-4-methylsulfanylbutanoyl]amino]propanoyl]amino]hexanoyl]amino]propanoyl]amino]-5-oxopentanoic acid;2,2,2-trifluoroacetic acid |
Source
|
---|---|---|
Source | PubChem | |
URL | https://pubchem.ncbi.nlm.nih.gov | |
Description | Data deposited in or computed by PubChem | |
InChI |
InChI=1S/C152H251N43O47S2.C2HF3O2/c1-72(2)60-102(145(237)195-110(69-113(157)198)150(242)177-90(34-29-57-165-152(161)162)134(226)189-106(64-76(9)10)146(238)193-107(65-77(11)12)147(239)192-105(63-75(7)8)144(236)183-98(42-51-119(209)210)138(230)180-93(37-46-114(199)200)129(221)167-79(15)121(158)213)187-126(218)81(17)168-122(214)80(16)169-130(222)91(35-44-111(155)196)178-136(228)95(39-48-116(203)204)174-125(217)84(20)171-131(223)94(38-47-115(201)202)181-135(227)92(36-45-112(156)197)179-137(229)96(40-49-117(205)206)175-124(216)83(19)170-128(220)88(32-26-27-55-153)173-123(215)82(18)172-132(224)100(53-58-243-21)185-140(232)99(43-52-120(211)212)184-143(235)103(61-73(3)4)190-141(233)101(54-59-244-22)186-139(231)97(41-50-118(207)208)182-133(225)89(33-28-56-164-151(159)160)176-142(234)104(62-74(5)6)191-148(240)108(66-78(13)14)194-149(241)109(68-86-70-163-71-166-86)188-127(219)87(154)67-85-30-24-23-25-31-85;3-2(4,5)1(6)7/h23-25,30-31,70-84,87-110H,26-29,32-69,153-154H2,1-22H3,(H2,155,196)(H2,156,197)(H2,157,198)(H2,158,213)(H,163,166)(H,167,221)(H,168,214)(H,169,222)(H,170,220)(H,171,223)(H,172,224)(H,173,215)(H,174,217)(H,175,216)(H,176,234)(H,177,242)(H,178,228)(H,179,229)(H,180,230)(H,181,227)(H,182,225)(H,183,236)(H,184,235)(H,185,232)(H,186,231)(H,187,218)(H,188,219)(H,189,226)(H,190,233)(H,191,240)(H,192,239)(H,193,238)(H,194,241)(H,195,237)(H,199,200)(H,201,202)(H,203,204)(H,205,206)(H,207,208)(H,209,210)(H,211,212)(H4,159,160,164)(H4,161,162,165);(H,6,7)/t79-,80-,81-,82-,83-,84-,87-,88-,89-,90-,91-,92-,93-,94-,95-,96-,97-,98-,99-,100-,101-,102-,103-,104-,105-,106-,107-,108-,109-,110-;/m0./s1 |
Source
|
Source | PubChem | |
URL | https://pubchem.ncbi.nlm.nih.gov | |
Description | Data deposited in or computed by PubChem | |
InChI Key |
VIVMEIHCWPNWJW-QFQWUVTISA-N |
Source
|
Source | PubChem | |
URL | https://pubchem.ncbi.nlm.nih.gov | |
Description | Data deposited in or computed by PubChem | |
Canonical SMILES |
CC(C)CC(C(=O)NC(CC(=O)N)C(=O)NC(CCCNC(=N)N)C(=O)NC(CC(C)C)C(=O)NC(CC(C)C)C(=O)NC(CC(C)C)C(=O)NC(CCC(=O)O)C(=O)NC(CCC(=O)O)C(=O)NC(C)C(=O)N)NC(=O)C(C)NC(=O)C(C)NC(=O)C(CCC(=O)N)NC(=O)C(CCC(=O)O)NC(=O)C(C)NC(=O)C(CCC(=O)O)NC(=O)C(CCC(=O)N)NC(=O)C(CCC(=O)O)NC(=O)C(C)NC(=O)C(CCCCN)NC(=O)C(C)NC(=O)C(CCSC)NC(=O)C(CCC(=O)O)NC(=O)C(CC(C)C)NC(=O)C(CCSC)NC(=O)C(CCC(=O)O)NC(=O)C(CCCNC(=N)N)NC(=O)C(CC(C)C)NC(=O)C(CC(C)C)NC(=O)C(CC1=CNC=N1)NC(=O)C(CC2=CC=CC=C2)N.C(=O)(C(F)(F)F)O |
Source
|
Source | PubChem | |
URL | https://pubchem.ncbi.nlm.nih.gov | |
Description | Data deposited in or computed by PubChem | |
Isomeric SMILES |
C[C@@H](C(=O)N)NC(=O)[C@H](CCC(=O)O)NC(=O)[C@H](CCC(=O)O)NC(=O)[C@H](CC(C)C)NC(=O)[C@H](CC(C)C)NC(=O)[C@H](CC(C)C)NC(=O)[C@H](CCCNC(=N)N)NC(=O)[C@H](CC(=O)N)NC(=O)[C@H](CC(C)C)NC(=O)[C@H](C)NC(=O)[C@H](C)NC(=O)[C@H](CCC(=O)N)NC(=O)[C@H](CCC(=O)O)NC(=O)[C@H](C)NC(=O)[C@H](CCC(=O)O)NC(=O)[C@H](CCC(=O)N)NC(=O)[C@H](CCC(=O)O)NC(=O)[C@H](C)NC(=O)[C@H](CCCCN)NC(=O)[C@H](C)NC(=O)[C@H](CCSC)NC(=O)[C@H](CCC(=O)O)NC(=O)[C@H](CC(C)C)NC(=O)[C@H](CCSC)NC(=O)[C@H](CCC(=O)O)NC(=O)[C@H](CCCNC(=N)N)NC(=O)[C@H](CC(C)C)NC(=O)[C@H](CC(C)C)NC(=O)[C@H](CC1=CNC=N1)NC(=O)[C@H](CC2=CC=CC=C2)N.C(=O)(C(F)(F)F)O |
Source
|
Source | PubChem | |
URL | https://pubchem.ncbi.nlm.nih.gov | |
Description | Data deposited in or computed by PubChem | |
Molecular Formula |
C154H252F3N43O49S2 |
Source
|
Source | PubChem | |
URL | https://pubchem.ncbi.nlm.nih.gov | |
Description | Data deposited in or computed by PubChem | |
Molecular Weight |
3611.0 g/mol |
Source
|
Source | PubChem | |
URL | https://pubchem.ncbi.nlm.nih.gov | |
Description | Data deposited in or computed by PubChem | |
Haftungsausschluss und Informationen zu In-Vitro-Forschungsprodukten
Bitte beachten Sie, dass alle Artikel und Produktinformationen, die auf BenchChem präsentiert werden, ausschließlich zu Informationszwecken bestimmt sind. Die auf BenchChem zum Kauf angebotenen Produkte sind speziell für In-vitro-Studien konzipiert, die außerhalb lebender Organismen durchgeführt werden. In-vitro-Studien, abgeleitet von dem lateinischen Begriff "in Glas", beinhalten Experimente, die in kontrollierten Laborumgebungen unter Verwendung von Zellen oder Geweben durchgeführt werden. Es ist wichtig zu beachten, dass diese Produkte nicht als Arzneimittel oder Medikamente eingestuft sind und keine Zulassung der FDA für die Vorbeugung, Behandlung oder Heilung von medizinischen Zuständen, Beschwerden oder Krankheiten erhalten haben. Wir müssen betonen, dass jede Form der körperlichen Einführung dieser Produkte in Menschen oder Tiere gesetzlich strikt untersagt ist. Es ist unerlässlich, sich an diese Richtlinien zu halten, um die Einhaltung rechtlicher und ethischer Standards in Forschung und Experiment zu gewährleisten.