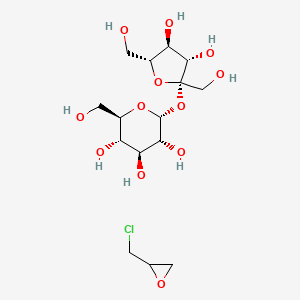
Polysucrose 400
Übersicht
Beschreibung
Polysucrose 400, also known as Ficoll 400, is a nonionic synthetic polymer of sucrose . It is a synthetic high molecular weight polymer made by the copolymerization of sucrose and epichlorohydrin . The molecules have a branched structure with a high content of hydroxyl groups, which gives it good solubility in aqueous solutions . It is used for the density gradient separation of cells, cell membranes, and virus cells .
Synthesis Analysis
Polysucrose 400 is synthesized by the copolymerization of sucrose and epichlorohydrin . The resulting molecules have a branched structure with a high content of hydroxyl groups .Molecular Structure Analysis
The molecules of Polysucrose 400 have a branched structure with a high content of hydroxyl groups . This structure gives the polymer good solubility in aqueous solutions .Physical And Chemical Properties Analysis
Polysucrose 400 is a solid at room temperature and is soluble in water up to 100 mg/ml . It has a molecular weight of 350000-500000 .Wissenschaftliche Forschungsanwendungen
Cell Separation and Organelle Isolation
Polysucrose 400 is used for the density gradient separation of cells, cell membranes, and virus cells . It can also be used to isolate different organelles .
Purification of Human Motile Spermatozoa
Polysucrose has been used in a study to develop a new method for the purification of human motile spermatozoa .
Antiproliferative and Antitumor Activities
Polysucrose has also been used in a study that investigated the antiproliferative and antitumor activities of D-reverse peptides .
Stabilizing Agent in Protein Solutions
Polysucrose 400 may be used as a stabilizing agent in protein solutions . This helps to maintain the structural integrity of proteins in solution, which is crucial for many biochemical and biophysical studies.
Immunologically Inert Carrier
Polysucrose 400 can function as an immunologically inert carrier for low molecular weight haptens in immunological studies . This allows for the study of immune responses to small molecules that would otherwise be ignored by the immune system.
Reduction of Non-Specific Binding
Polysucrose 400 is also used to reduce non-specific binding of labelled probes to nitrocellulose membranes during nucleic acid hybridization . This helps to increase the specificity and accuracy of these hybridization experiments.
7. Loading of Nucleic Acids for Electrophoresis Polysucrose 400 simplifies the loading of nucleic acids into the sample wells of agarose gels for electrophoresis . This makes the process of preparing samples for gel electrophoresis more efficient and reliable.
Wirkmechanismus
- While it doesn’t have specific protein targets, it plays a crucial role in density gradient separation of cells, cell membranes, and virus particles. Additionally, it can be used to isolate different organelles .
Target of Action
Mode of Action
Zukünftige Richtungen
Polysucrose 400 is widely used in biological research, particularly for the separation of cells and organelles . It may also be used as a direct replacement for Ficoll in common protocols . Its future use will likely continue to be in these areas, and potentially expand as new applications are discovered.
Eigenschaften
IUPAC Name |
2-(chloromethyl)oxirane;(2R,3R,4S,5S,6R)-2-[(2S,3S,4S,5R)-3,4-dihydroxy-2,5-bis(hydroxymethyl)oxolan-2-yl]oxy-6-(hydroxymethyl)oxane-3,4,5-triol | |
---|---|---|
Source | PubChem | |
URL | https://pubchem.ncbi.nlm.nih.gov | |
Description | Data deposited in or computed by PubChem | |
InChI |
InChI=1S/C12H22O11.C3H5ClO/c13-1-4-6(16)8(18)9(19)11(21-4)23-12(3-15)10(20)7(17)5(2-14)22-12;4-1-3-2-5-3/h4-11,13-20H,1-3H2;3H,1-2H2/t4-,5-,6-,7-,8+,9-,10+,11-,12+;/m1./s1 | |
Source | PubChem | |
URL | https://pubchem.ncbi.nlm.nih.gov | |
Description | Data deposited in or computed by PubChem | |
InChI Key |
IACFXVUNKCXYJM-AKSHDPDZSA-N | |
Source | PubChem | |
URL | https://pubchem.ncbi.nlm.nih.gov | |
Description | Data deposited in or computed by PubChem | |
Canonical SMILES |
C1C(O1)CCl.C(C1C(C(C(C(O1)OC2(C(C(C(O2)CO)O)O)CO)O)O)O)O | |
Source | PubChem | |
URL | https://pubchem.ncbi.nlm.nih.gov | |
Description | Data deposited in or computed by PubChem | |
Isomeric SMILES |
C1C(O1)CCl.C([C@@H]1[C@H]([C@@H]([C@H]([C@H](O1)O[C@]2([C@H]([C@@H]([C@H](O2)CO)O)O)CO)O)O)O)O | |
Source | PubChem | |
URL | https://pubchem.ncbi.nlm.nih.gov | |
Description | Data deposited in or computed by PubChem | |
Molecular Formula |
C15H27ClO12 | |
Source | PubChem | |
URL | https://pubchem.ncbi.nlm.nih.gov | |
Description | Data deposited in or computed by PubChem | |
Related CAS |
26873-85-8 | |
Record name | Epichlorohydrin-sucrose copolymer | |
Source | CAS Common Chemistry | |
URL | https://commonchemistry.cas.org/detail?cas_rn=26873-85-8 | |
Description | CAS Common Chemistry is an open community resource for accessing chemical information. Nearly 500,000 chemical substances from CAS REGISTRY cover areas of community interest, including common and frequently regulated chemicals, and those relevant to high school and undergraduate chemistry classes. This chemical information, curated by our expert scientists, is provided in alignment with our mission as a division of the American Chemical Society. | |
Explanation | The data from CAS Common Chemistry is provided under a CC-BY-NC 4.0 license, unless otherwise stated. | |
Molecular Weight |
434.82 g/mol | |
Source | PubChem | |
URL | https://pubchem.ncbi.nlm.nih.gov | |
Description | Data deposited in or computed by PubChem | |
Physical Description |
White lyophilized powder; [Sigma-Aldrich MSDS] | |
Record name | alpha-D-Glucopyranoside, beta-D-fructofuranosyl, polymer with 2-(chloromethyl)oxirane | |
Source | Haz-Map, Information on Hazardous Chemicals and Occupational Diseases | |
URL | https://haz-map.com/Agents/11729 | |
Description | Haz-Map® is an occupational health database designed for health and safety professionals and for consumers seeking information about the adverse effects of workplace exposures to chemical and biological agents. | |
Explanation | Copyright (c) 2022 Haz-Map(R). All rights reserved. Unless otherwise indicated, all materials from Haz-Map are copyrighted by Haz-Map(R). No part of these materials, either text or image may be used for any purpose other than for personal use. Therefore, reproduction, modification, storage in a retrieval system or retransmission, in any form or by any means, electronic, mechanical or otherwise, for reasons other than personal use, is strictly prohibited without prior written permission. | |
CAS RN |
26873-85-8 | |
Record name | .alpha.-D-Glucopyranoside, .beta.-D-fructofuranosyl, polymer with 2-(chloromethyl)oxirane | |
Source | EPA Chemicals under the TSCA | |
URL | https://www.epa.gov/chemicals-under-tsca | |
Description | EPA Chemicals under the Toxic Substances Control Act (TSCA) collection contains information on chemicals and their regulations under TSCA, including non-confidential content from the TSCA Chemical Substance Inventory and Chemical Data Reporting. | |
Record name | α-D-Glucopyranoside, β-D-fructofuranosyl, polymer with 2-(chloromethyl)oxirane | |
Source | European Chemicals Agency (ECHA) | |
URL | https://echa.europa.eu/substance-information/-/substanceinfo/100.126.925 | |
Description | The European Chemicals Agency (ECHA) is an agency of the European Union which is the driving force among regulatory authorities in implementing the EU's groundbreaking chemicals legislation for the benefit of human health and the environment as well as for innovation and competitiveness. | |
Explanation | Use of the information, documents and data from the ECHA website is subject to the terms and conditions of this Legal Notice, and subject to other binding limitations provided for under applicable law, the information, documents and data made available on the ECHA website may be reproduced, distributed and/or used, totally or in part, for non-commercial purposes provided that ECHA is acknowledged as the source: "Source: European Chemicals Agency, http://echa.europa.eu/". Such acknowledgement must be included in each copy of the material. ECHA permits and encourages organisations and individuals to create links to the ECHA website under the following cumulative conditions: Links can only be made to webpages that provide a link to the Legal Notice page. | |
Q & A
Q1: What is the role of Polysucrose 400 in cell separation procedures?
A1: Polysucrose 400 is a key component in density gradient centrifugation media used for isolating specific cell populations []. In conjunction with sodium diatrizoate, it forms a density gradient within a centrifuge tube. When a biological sample like blood is layered on top and centrifuged, cells separate based on their density, allowing researchers to collect specific cell types for downstream analyses [].
Q2: How does Polysucrose 400 impact the analysis of Matrix Metalloproteinases (MMPs)?
A2: While Polysucrose 400 itself doesn't directly influence MMP concentrations or their isoform profiles [], the complete cell separation media containing Polysucrose 400 can lead to an observed increase in the concentration of all MMPs in the collected plasma fraction []. This effect is likely due to the differential release of MMPs from platelets and leukocytes, highlighting the importance of considering cell separation media composition when analyzing MMPs [].
Q3: Does Polysucrose 400 influence the protective properties of sucrose in vitrification solutions?
A3: While not directly addressed in the provided research, it's important to note that Polysucrose 400, due to its polymeric structure, exhibits different properties compared to monomeric sucrose []. While monomeric sucrose displays a positive correlation between increased glass transition temperature and protection during drying, this correlation becomes inverse for Polysucrose 400 []. This suggests that the size of sucrose polymers significantly influences the protective properties within vitrification solutions.
Haftungsausschluss und Informationen zu In-Vitro-Forschungsprodukten
Bitte beachten Sie, dass alle Artikel und Produktinformationen, die auf BenchChem präsentiert werden, ausschließlich zu Informationszwecken bestimmt sind. Die auf BenchChem zum Kauf angebotenen Produkte sind speziell für In-vitro-Studien konzipiert, die außerhalb lebender Organismen durchgeführt werden. In-vitro-Studien, abgeleitet von dem lateinischen Begriff "in Glas", beinhalten Experimente, die in kontrollierten Laborumgebungen unter Verwendung von Zellen oder Geweben durchgeführt werden. Es ist wichtig zu beachten, dass diese Produkte nicht als Arzneimittel oder Medikamente eingestuft sind und keine Zulassung der FDA für die Vorbeugung, Behandlung oder Heilung von medizinischen Zuständen, Beschwerden oder Krankheiten erhalten haben. Wir müssen betonen, dass jede Form der körperlichen Einführung dieser Produkte in Menschen oder Tiere gesetzlich strikt untersagt ist. Es ist unerlässlich, sich an diese Richtlinien zu halten, um die Einhaltung rechtlicher und ethischer Standards in Forschung und Experiment zu gewährleisten.