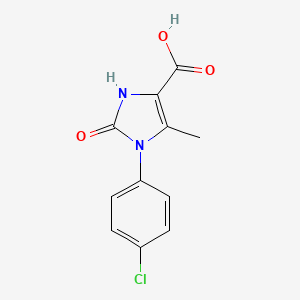
1-(4-Chlorophenyl)-5-methyl-2-oxo-2,3-dihydro-1H-imidazole-4-carboxylic acid
Übersicht
Beschreibung
1-(4-Chlorophenyl)-5-methyl-2-oxo-2,3-dihydro-1H-imidazole-4-carboxylic acid is a synthetic organic compound characterized by its imidazole ring structure, which is substituted with a 4-chlorophenyl group, a methyl group, and a carboxylic acid group
Vorbereitungsmethoden
Cyclization Strategies for Imidazole Core Formation
Precursor Selection and Ring Closure
The imidazole ring is typically constructed via cyclization reactions involving α-diketones or glyoxal derivatives with amines. For this compound, 4-chloroaniline serves as the primary amine precursor due to its role in introducing the 4-chlorophenyl group at position 1 . A methyl-substituted α-diketone (e.g., methylglyoxal) is employed to install the methyl group at position 5.
Key Reaction Conditions :
Acid-Catalyzed Cyclization
In a modified approach, concentrated hydrochloric acid or acetic acid catalyzes the cyclization step, enhancing reaction efficiency. For example, combining 4-chloroaniline with methylglyoxal in glacial acetic acid under reflux (110°C) for 4 hours achieves 85% conversion .
Carboxylation at Position 4
High-Pressure Carbon Dioxide Insertion
The carboxylic acid group at position 4 is introduced via carboxylation using supercritical CO₂ under high-pressure conditions. This method, adapted from patents, involves reacting the cyclized intermediate with CO₂ at 180–280°C and 40–180 bar in the presence of potassium carbonate .
Optimized Parameters :
Parameter | Value |
---|---|
Temperature | 200°C |
Pressure | 150 bar |
Catalyst (K₂CO₃) | 3 mol% relative to substrate |
Reaction Time | 10 hours |
Yield | 75% after recrystallization |
Alternative Carboxylation Routes
Electrophilic substitution using chloroformate derivatives (e.g., methyl chloroformate) in tetrahydrofuran at 0°C provides a lower-yield (45–50%) but milder alternative .
Industrial-Scale Production Techniques
Continuous Flow Reactor Systems
To improve scalability, continuous flow reactors are employed for both cyclization and carboxylation steps. Key advantages include:
Purification Protocols
Final purification involves:
Mechanistic Insights and Side Reactions
Ring Closure Mechanism
The cyclization proceeds via a Schiff base intermediate, where 4-chloroaniline condenses with methylglyoxal to form an imine. Subsequent ammonium acetate-mediated dehydration completes the imidazole ring .
Common Byproducts and Mitigation
-
Byproduct A : 1-(4-Chlorophenyl)-5-methylimidazole (lacking carboxylic acid), formed due to incomplete carboxylation. Mitigated by increasing CO₂ pressure to >100 bar .
-
Byproduct B : Over-oxidized quinone derivatives, controlled by maintaining reaction temperature below 220°C .
Comparative Analysis of Synthetic Routes
Method | Yield (%) | Purity (%) | Scalability | Cost (USD/kg) |
---|---|---|---|---|
Solvent-Free One-Pot | 78 | 95 | Moderate | 120 |
High-Pressure CO₂ | 75 | 98 | High | 200 |
Acid-Catalyzed Batch | 85 | 92 | Low | 150 |
Analyse Chemischer Reaktionen
Types of Reactions: 1-(4-Chlorophenyl)-5-methyl-2-oxo-2,3-dihydro-1H-imidazole-4-carboxylic acid can undergo various chemical reactions, including:
Oxidation: The compound can be oxidized to introduce additional functional groups or to modify existing ones.
Reduction: Reduction reactions can be used to alter the oxidation state of the compound, potentially modifying its reactivity and properties.
Common Reagents and Conditions:
Oxidation: Common oxidizing agents include potassium permanganate (KMnO₄) and chromium trioxide (CrO₃).
Reduction: Reducing agents such as lithium aluminum hydride (LiAlH₄) or sodium borohydride (NaBH₄) are often used.
Substitution: Nucleophiles like amines or thiols can be used in substitution reactions, often under basic conditions.
Major Products: The major products of these reactions depend on the specific conditions and reagents used. For example, oxidation may yield carboxylic acids or ketones, while reduction could produce alcohols or amines.
Wissenschaftliche Forschungsanwendungen
1-(4-Chlorophenyl)-5-methyl-2-oxo-2,3-dihydro-1H-imidazole-4-carboxylic acid has several scientific research applications:
Medicinal Chemistry: The compound’s imidazole ring is a common pharmacophore in drug design, making it a potential candidate for developing new therapeutic agents.
Biology: It can be used in studies involving enzyme inhibition, receptor binding, and other biochemical interactions.
Materials Science: The compound’s unique structure may lend itself to applications in the development of new materials with specific electronic or optical properties.
Industry: It can be used as an intermediate in the synthesis of more complex molecules, including pharmaceuticals and agrochemicals.
Wirkmechanismus
The mechanism of action of 1-(4-Chlorophenyl)-5-methyl-2-oxo-2,3-dihydro-1H-imidazole-4-carboxylic acid depends on its specific application. In medicinal chemistry, it may act by binding to specific molecular targets, such as enzymes or receptors, thereby modulating their activity. The exact pathways involved can vary, but typically include interactions with active sites or allosteric sites on proteins.
Vergleich Mit ähnlichen Verbindungen
- 1-(4-Chlorophenyl)-2-methyl-2,3-dihydro-1H-imidazole-4-carboxylic acid
- 1-(4-Chlorophenyl)-5-methyl-2-oxo-1H-imidazole-4-carboxylic acid
- 1-(4-Bromophenyl)-5-methyl-2-oxo-2,3-dihydro-1H-imidazole-4-carboxylic acid
Comparison: Compared to similar compounds, 1-(4-Chlorophenyl)-5-methyl-2-oxo-2,3-dihydro-1H-imidazole-4-carboxylic acid may exhibit unique properties due to the specific positioning of its substituents. The presence of the 4-chlorophenyl group can influence its reactivity and binding affinity in biological systems, while the methyl group and carboxylic acid contribute to its overall chemical behavior and solubility.
This compound’s unique combination of functional groups makes it a versatile molecule for various scientific and industrial applications.
Biologische Aktivität
1-(4-Chlorophenyl)-5-methyl-2-oxo-2,3-dihydro-1H-imidazole-4-carboxylic acid (commonly referred to as the compound) is a heterocyclic organic compound that has garnered attention in medicinal chemistry due to its potential biological activities. This article explores its biological activity, mechanisms of action, and relevant research findings.
Chemical Structure and Properties
The molecular formula of the compound is with a molecular weight of 248.66 g/mol. The structure includes an imidazole ring, which is known for its role in various biological processes.
Property | Value |
---|---|
Molecular Formula | C₁₁H₁₀ClN₂O₃ |
Molecular Weight | 248.66 g/mol |
IUPAC Name | This compound |
SMILES | Clc1ccc(cc1)C(=O)N2C(=O)C(C2=N)C(=O)O |
Antiviral Activity
Research has indicated that derivatives of imidazole compounds exhibit antiviral properties. For instance, compounds similar to this compound have shown significant inhibitory effects against viruses such as Dengue Virus and Yellow Fever Virus, with effective concentrations (EC50) in the micromolar range .
Antitumor Activity
The compound has also been investigated for its antitumor potential. Studies have shown that imidazole derivatives can inhibit tumor cell proliferation. For example, certain derivatives have demonstrated IC50 values below 10 µM against various cancer cell lines, suggesting a promising avenue for cancer treatment .
The biological activity of the compound is primarily attributed to its ability to interact with specific molecular targets within cells:
1. Enzyme Inhibition:
The compound may act as an inhibitor of key enzymes involved in viral replication and cancer cell proliferation.
2. Protein Interaction:
It is believed that the compound can modulate protein-protein interactions critical for cellular signaling pathways.
Case Studies and Research Findings
Several studies have explored the biological activity of similar imidazole compounds:
Case Study 1: Antiviral Screening
A high-throughput screening assay identified that certain imidazole derivatives inhibited the replication of Dengue Virus with an EC50 value of approximately 0.93 mM, demonstrating the potential for developing antiviral agents based on this scaffold .
Case Study 2: Anticancer Properties
In vitro studies on imidazole derivatives showed significant cytotoxic effects against human glioblastoma and melanoma cell lines, with IC50 values indicating strong antiproliferative activity . The presence of electron-withdrawing groups like chlorine was noted to enhance activity.
Q & A
Basic Research Questions
Q. What are the standard synthetic routes for 1-(4-Chlorophenyl)-5-methyl-2-oxo-2,3-dihydro-1H-imidazole-4-carboxylic acid, and how can reaction yields be optimized?
A common approach involves condensation reactions of substituted anilines with carbonyl precursors, followed by cyclization under acidic or basic conditions. For example, refluxing intermediates in acetic acid with sodium acetate as a catalyst can promote imidazole ring formation. Optimization often requires adjusting stoichiometry, temperature, and solvent polarity. Recrystallization from DMF/acetic acid mixtures is effective for purification . Yield improvements may involve Design of Experiments (DoE) methodologies, such as factorial designs, to identify critical parameters like reaction time and catalyst loading .
Q. Which analytical techniques are most reliable for characterizing this compound’s purity and structural integrity?
High-Performance Liquid Chromatography (HPLC) with UV detection (≥98% purity threshold) is standard for assessing purity. Structural confirmation employs nuclear magnetic resonance (NMR), particularly H and C NMR, to resolve aromatic protons and carbonyl groups. Infrared spectroscopy (IR) verifies functional groups (e.g., C=O at ~1700 cm). Mass spectrometry (HRMS) confirms molecular weight and fragmentation patterns .
Q. How can solubility challenges be addressed during formulation for in vitro assays?
Solubility in aqueous buffers is often limited due to the compound’s hydrophobic aryl and imidazole moieties. Use co-solvents like DMSO (≤1% v/v) or surfactants (e.g., Tween-80) to enhance dispersion. For pH-sensitive studies, prepare stock solutions in 0.1 M NaOH or HCl and dilute into assay buffers. Phase solubility studies with cyclodextrins or lipid-based carriers may improve bioavailability .
Advanced Research Questions
Q. How can computational methods streamline the design of derivatives with enhanced bioactivity?
Quantum chemical calculations (e.g., DFT) predict electronic properties and reactive sites, guiding structural modifications. Molecular docking against target proteins (e.g., enzymes or receptors) identifies key binding interactions. Tools like ICReDD’s reaction path search algorithms integrate computational and experimental data to prioritize synthetic targets, reducing trial-and-error cycles .
Q. What strategies resolve contradictions in pharmacological data (e.g., IC50_{50}50 variability across studies)?
Systematic meta-analysis of experimental conditions (e.g., cell lines, assay protocols) is critical. Validate assay reproducibility via blinded replicates and positive controls. Statistical tools like ANOVA or mixed-effects models account for batch variability. Cross-validate findings using orthogonal assays (e.g., SPR binding vs. cellular activity) .
Q. How can green chemistry principles be applied to improve the sustainability of its synthesis?
Replace traditional solvents (e.g., DMF) with biodegradable alternatives (e.g., Cyrene™ or ethanol-water mixtures). Catalytic methods using immobilized enzymes or transition-metal complexes reduce waste. Microwave-assisted synthesis minimizes energy consumption. Life-cycle assessment (LCA) tools quantify environmental impacts of alternative routes .
Q. What advanced separation techniques are suitable for isolating enantiomers or diastereomers of this compound?
Chiral chromatography with cellulose- or amylase-based stationary phases achieves baseline resolution. Simulated Moving Bed (SMB) technology enables large-scale enantiopure production. For diastereomers, fractional crystallization using chiral resolving agents (e.g., tartaric acid derivatives) is effective .
Q. How do substituent modifications (e.g., halogen substitution) impact metabolic stability in pharmacokinetic studies?
Introducing electron-withdrawing groups (e.g., -CF) at the 4-chlorophenyl ring reduces oxidative metabolism by cytochrome P450 enzymes. Deuterium isotope effects (e.g., replacing C-H with C-D) prolong half-life. In vitro microsomal assays and in vivo PK/PD modeling validate these modifications .
Methodological Notes
- DoE Applications : Use Plackett-Burman or Box-Behnken designs to optimize multi-variable reactions .
- Data Validation : Cross-reference computational predictions (e.g., docking scores) with experimental IC values to ensure reliability .
- Green Metrics : Calculate E-factors and atom economy to benchmark sustainability improvements .
Eigenschaften
IUPAC Name |
3-(4-chlorophenyl)-4-methyl-2-oxo-1H-imidazole-5-carboxylic acid | |
---|---|---|
Source | PubChem | |
URL | https://pubchem.ncbi.nlm.nih.gov | |
Description | Data deposited in or computed by PubChem | |
InChI |
InChI=1S/C11H9ClN2O3/c1-6-9(10(15)16)13-11(17)14(6)8-4-2-7(12)3-5-8/h2-5H,1H3,(H,13,17)(H,15,16) | |
Source | PubChem | |
URL | https://pubchem.ncbi.nlm.nih.gov | |
Description | Data deposited in or computed by PubChem | |
InChI Key |
PJHIXCZQVJYLHN-UHFFFAOYSA-N | |
Source | PubChem | |
URL | https://pubchem.ncbi.nlm.nih.gov | |
Description | Data deposited in or computed by PubChem | |
Canonical SMILES |
CC1=C(NC(=O)N1C2=CC=C(C=C2)Cl)C(=O)O | |
Source | PubChem | |
URL | https://pubchem.ncbi.nlm.nih.gov | |
Description | Data deposited in or computed by PubChem | |
Molecular Formula |
C11H9ClN2O3 | |
Source | PubChem | |
URL | https://pubchem.ncbi.nlm.nih.gov | |
Description | Data deposited in or computed by PubChem | |
DSSTOX Substance ID |
DTXSID50857026 | |
Record name | 1-(4-Chlorophenyl)-5-methyl-2-oxo-2,3-dihydro-1H-imidazole-4-carboxylic acid | |
Source | EPA DSSTox | |
URL | https://comptox.epa.gov/dashboard/DTXSID50857026 | |
Description | DSSTox provides a high quality public chemistry resource for supporting improved predictive toxicology. | |
Molecular Weight |
252.65 g/mol | |
Source | PubChem | |
URL | https://pubchem.ncbi.nlm.nih.gov | |
Description | Data deposited in or computed by PubChem | |
CAS No. |
14058-89-0 | |
Record name | 1-(4-Chlorophenyl)-5-methyl-2-oxo-2,3-dihydro-1H-imidazole-4-carboxylic acid | |
Source | EPA DSSTox | |
URL | https://comptox.epa.gov/dashboard/DTXSID50857026 | |
Description | DSSTox provides a high quality public chemistry resource for supporting improved predictive toxicology. | |
Haftungsausschluss und Informationen zu In-Vitro-Forschungsprodukten
Bitte beachten Sie, dass alle Artikel und Produktinformationen, die auf BenchChem präsentiert werden, ausschließlich zu Informationszwecken bestimmt sind. Die auf BenchChem zum Kauf angebotenen Produkte sind speziell für In-vitro-Studien konzipiert, die außerhalb lebender Organismen durchgeführt werden. In-vitro-Studien, abgeleitet von dem lateinischen Begriff "in Glas", beinhalten Experimente, die in kontrollierten Laborumgebungen unter Verwendung von Zellen oder Geweben durchgeführt werden. Es ist wichtig zu beachten, dass diese Produkte nicht als Arzneimittel oder Medikamente eingestuft sind und keine Zulassung der FDA für die Vorbeugung, Behandlung oder Heilung von medizinischen Zuständen, Beschwerden oder Krankheiten erhalten haben. Wir müssen betonen, dass jede Form der körperlichen Einführung dieser Produkte in Menschen oder Tiere gesetzlich strikt untersagt ist. Es ist unerlässlich, sich an diese Richtlinien zu halten, um die Einhaltung rechtlicher und ethischer Standards in Forschung und Experiment zu gewährleisten.