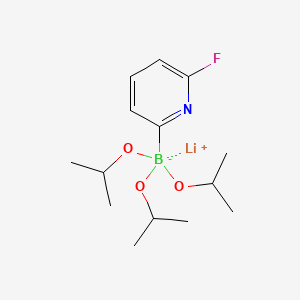
Lithium-Triisopropyl-2-(6-Fluorpyridyl)borat
- Klicken Sie auf QUICK INQUIRY, um ein Angebot von unserem Expertenteam zu erhalten.
- Mit qualitativ hochwertigen Produkten zu einem WETTBEWERBSFÄHIGEN Preis können Sie sich mehr auf Ihre Forschung konzentrieren.
Übersicht
Beschreibung
Lithium triisopropyl 2-(6-fluoropyridyl)borate is a chemical compound with the molecular formula C14H24BFLiNO3. It is known for its unique structure, which includes a lithium ion coordinated to a borate group and a fluoropyridyl moiety.
Wissenschaftliche Forschungsanwendungen
Lithium triisopropyl 2-(6-fluoropyridyl)borate has several scientific research applications, including:
Organic Synthesis: It is used as a reagent in the synthesis of complex organic molecules, particularly in the formation of carbon-carbon and carbon-heteroatom bonds.
Materials Science: The compound is investigated for its potential use in the development of new materials, such as polymers and catalysts.
Medicinal Chemistry: Researchers explore its use in the synthesis of pharmaceutical intermediates and active pharmaceutical ingredients.
Biological Studies: It is used in the study of biological systems, particularly in the development of probes and imaging agents.
Safety and Hazards
Vorbereitungsmethoden
Synthetic Routes and Reaction Conditions
The synthesis of lithium triisopropyl 2-(6-fluoropyridyl)borate typically involves the reaction of triisopropyl borate with 2-(6-fluoropyridyl)lithium. The reaction is carried out under an inert atmosphere, such as nitrogen or argon, to prevent moisture and oxygen from interfering with the reaction. The reaction mixture is usually stirred at low temperatures to ensure the formation of the desired product .
Industrial Production Methods
While specific industrial production methods for lithium triisopropyl 2-(6-fluoropyridyl)borate are not widely documented, the general approach involves scaling up the laboratory synthesis procedures. This includes optimizing reaction conditions, such as temperature, pressure, and solvent choice, to achieve higher yields and purity on an industrial scale .
Analyse Chemischer Reaktionen
Types of Reactions
Lithium triisopropyl 2-(6-fluoropyridyl)borate can undergo various types of chemical reactions, including:
Substitution Reactions: The fluoropyridyl group can participate in nucleophilic substitution reactions, where the fluorine atom is replaced by other nucleophiles.
Oxidation and Reduction Reactions: The borate group can be involved in redox reactions, where it can either gain or lose electrons.
Common Reagents and Conditions
Common reagents used in reactions with lithium triisopropyl 2-(6-fluoropyridyl)borate include:
Nucleophiles: Such as amines, thiols, and alkoxides for substitution reactions.
Oxidizing Agents: Such as hydrogen peroxide or potassium permanganate for oxidation reactions.
Reducing Agents: Such as lithium aluminum hydride or sodium borohydride for reduction reactions.
Major Products Formed
The major products formed from reactions involving lithium triisopropyl 2-(6-fluoropyridyl)borate depend on the specific reaction conditions and reagents used. For example, nucleophilic substitution reactions can yield various substituted pyridylborates, while oxidation reactions can produce boronic acids or esters .
Wirkmechanismus
The mechanism of action of lithium triisopropyl 2-(6-fluoropyridyl)borate involves its ability to act as a nucleophile or electrophile in various chemical reactions. The lithium ion can coordinate with other molecules, facilitating the formation of new bonds. The fluoropyridyl group can participate in substitution reactions, while the borate group can undergo redox reactions. These interactions enable the compound to exert its effects in different chemical and biological systems .
Vergleich Mit ähnlichen Verbindungen
Similar Compounds
Lithium triisopropyl borate: Similar in structure but lacks the fluoropyridyl group.
Lithium 2-(6-fluoropyridyl)borate: Similar but without the triisopropyl groups.
Lithium triisopropyl 2-pyridylborate: Similar but without the fluorine atom on the pyridyl ring.
Uniqueness
Lithium triisopropyl 2-(6-fluoropyridyl)borate is unique due to the presence of both the triisopropyl borate and fluoropyridyl groups. This combination imparts distinct chemical properties, such as enhanced reactivity and stability, making it valuable in various research applications .
Eigenschaften
IUPAC Name |
lithium;(6-fluoropyridin-2-yl)-tri(propan-2-yloxy)boranuide |
Source
|
---|---|---|
Source | PubChem | |
URL | https://pubchem.ncbi.nlm.nih.gov | |
Description | Data deposited in or computed by PubChem | |
InChI |
InChI=1S/C14H24BFNO3.Li/c1-10(2)18-15(19-11(3)4,20-12(5)6)13-8-7-9-14(16)17-13;/h7-12H,1-6H3;/q-1;+1 |
Source
|
Source | PubChem | |
URL | https://pubchem.ncbi.nlm.nih.gov | |
Description | Data deposited in or computed by PubChem | |
InChI Key |
NYQSATBYBIKYSU-UHFFFAOYSA-N |
Source
|
Source | PubChem | |
URL | https://pubchem.ncbi.nlm.nih.gov | |
Description | Data deposited in or computed by PubChem | |
Canonical SMILES |
[Li+].[B-](C1=NC(=CC=C1)F)(OC(C)C)(OC(C)C)OC(C)C |
Source
|
Source | PubChem | |
URL | https://pubchem.ncbi.nlm.nih.gov | |
Description | Data deposited in or computed by PubChem | |
Molecular Formula |
C14H24BFLiNO3 |
Source
|
Source | PubChem | |
URL | https://pubchem.ncbi.nlm.nih.gov | |
Description | Data deposited in or computed by PubChem | |
Molecular Weight |
291.1 g/mol |
Source
|
Source | PubChem | |
URL | https://pubchem.ncbi.nlm.nih.gov | |
Description | Data deposited in or computed by PubChem | |
Haftungsausschluss und Informationen zu In-Vitro-Forschungsprodukten
Bitte beachten Sie, dass alle Artikel und Produktinformationen, die auf BenchChem präsentiert werden, ausschließlich zu Informationszwecken bestimmt sind. Die auf BenchChem zum Kauf angebotenen Produkte sind speziell für In-vitro-Studien konzipiert, die außerhalb lebender Organismen durchgeführt werden. In-vitro-Studien, abgeleitet von dem lateinischen Begriff "in Glas", beinhalten Experimente, die in kontrollierten Laborumgebungen unter Verwendung von Zellen oder Geweben durchgeführt werden. Es ist wichtig zu beachten, dass diese Produkte nicht als Arzneimittel oder Medikamente eingestuft sind und keine Zulassung der FDA für die Vorbeugung, Behandlung oder Heilung von medizinischen Zuständen, Beschwerden oder Krankheiten erhalten haben. Wir müssen betonen, dass jede Form der körperlichen Einführung dieser Produkte in Menschen oder Tiere gesetzlich strikt untersagt ist. Es ist unerlässlich, sich an diese Richtlinien zu halten, um die Einhaltung rechtlicher und ethischer Standards in Forschung und Experiment zu gewährleisten.