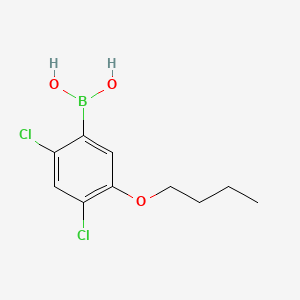
(5-Butoxy-2,4-dichlorophenyl)boronic acid
Übersicht
Beschreibung
(5-Butoxy-2,4-dichlorophenyl)boronic acid is an organoboron compound characterized by a phenyl ring substituted with a butoxy group (-O-C₄H₉) at position 5 and chlorine atoms at positions 2 and 3. This structure combines electron-withdrawing (chlorine) and electron-donating (butoxy) substituents, which influence its Lewis acidity, solubility, and reactivity. Boronic acids are widely used in Suzuki-Miyaura cross-coupling reactions, molecular sensing, and drug development due to their ability to form reversible covalent bonds with diols and interact with biological targets like proteases or tubulin .
Vorbereitungsmethoden
Synthetic Routes and Reaction Conditions
The synthesis of (5-Butoxy-2,4-dichlorophenyl)boronic acid typically involves the borylation of the corresponding aryl halide. One common method is the palladium-catalyzed borylation of 5-butoxy-2,4-dichlorobenzene using bis(pinacolato)diboron as the boron source . The reaction is usually carried out in the presence of a base such as potassium carbonate and a ligand like triphenylphosphine under an inert atmosphere.
Industrial Production Methods
While specific industrial production methods for this compound are not well-documented, the general approach involves scaling up the laboratory synthesis methods. This includes optimizing reaction conditions to improve yield and purity, as well as implementing continuous flow processes to enhance efficiency and safety.
Analyse Chemischer Reaktionen
Types of Reactions
(5-Butoxy-2,4-dichlorophenyl)boronic acid primarily undergoes reactions typical of boronic acids, including:
Suzuki-Miyaura Coupling: This reaction forms carbon-carbon bonds by coupling the boronic acid with an aryl or vinyl halide in the presence of a palladium catalyst.
Oxidation: Boronic acids can be oxidized to form phenols using hydrogen peroxide or other oxidizing agents.
Esterification: Reaction with alcohols to form boronate esters.
Common Reagents and Conditions
Suzuki-Miyaura Coupling: Palladium catalyst, base (e.g., potassium carbonate), ligand (e.g., triphenylphosphine), and an inert atmosphere.
Oxidation: Hydrogen peroxide or other oxidizing agents.
Esterification: Alcohols and acid catalysts.
Major Products
Suzuki-Miyaura Coupling: Biaryl compounds.
Oxidation: Phenols.
Esterification: Boronate esters.
Wissenschaftliche Forschungsanwendungen
(5-Butoxy-2,4-dichlorophenyl)boronic acid has several applications in scientific research:
Biology: Potential use in the development of boron-containing drugs due to its ability to form stable complexes with biomolecules.
Medicine: Investigated for its potential in boron neutron capture therapy (BNCT) for cancer treatment.
Industry: Utilized in the synthesis of advanced materials and polymers.
Wirkmechanismus
The mechanism of action of (5-Butoxy-2,4-dichlorophenyl)boronic acid in Suzuki-Miyaura coupling involves several key steps:
Oxidative Addition: The palladium catalyst inserts into the carbon-halogen bond of the aryl halide.
Transmetalation: The boronic acid transfers its aryl group to the palladium complex.
Reductive Elimination: The palladium complex forms the new carbon-carbon bond and regenerates the palladium catalyst.
Vergleich Mit ähnlichen Verbindungen
The following analysis compares (5-Butoxy-2,4-dichlorophenyl)boronic acid with structurally or functionally related boronic acids, focusing on physicochemical properties, biological activity, and applications.
Substituent Effects on Acidity (pKa) and Reactivity
The pKa of boronic acids determines their ability to form boronate esters with diols under physiological conditions. Electron-withdrawing groups (e.g., -Cl) lower pKa by stabilizing the conjugate base, while electron-donating groups (e.g., -OBut) increase pKa.
*Estimated based on substituent effects. The butoxy group likely raises pKa compared to purely electron-withdrawing substituents.
Key Insight : The butoxy group in this compound may reduce its reactivity toward diols under physiological conditions compared to 2,4-dichlorophenylboronic acid but improve stability in hydrophobic environments .
Solubility and Stability
Substituents critically affect solubility and stability in aqueous media:
*Predicted based on hydrophobic butoxy group.
Key Insight : The butoxy substituent may reduce aqueous solubility compared to hydroxylated analogs (e.g., 6-hydroxynaphthalen-2-yl boronic acid) but improve compatibility with lipid membranes .
Biologische Aktivität
(5-Butoxy-2,4-dichlorophenyl)boronic acid is a boronic acid derivative known for its potential biological activities. Its unique structure allows it to participate in various biochemical interactions, making it a subject of interest in medicinal chemistry and pharmacology. This article explores the biological activity of this compound, focusing on its mechanisms of action, therapeutic applications, and relevant research findings.
- Chemical Formula : C10H13BCl2O3
- Molar Mass : 262.93 g/mol
- CAS Number : 1256354-88-7
- Density : 1.30±0.1 g/cm³ (Predicted)
- Boiling Point : 403.9±55.0 °C (Predicted)
- pKa : 7.65±0.58 (Predicted)
These properties indicate that this compound is stable under various conditions, which is essential for its application in biological systems.
Boronic acids, including this compound, are known to interact with various biological targets through reversible covalent bonding with diols and other nucleophiles. This interaction can modulate enzyme activity and influence cellular signaling pathways.
- Inhibition of Enzymatic Activity : Boronic acids have been shown to inhibit enzymes such as hormone-sensitive lipase (HSL), which plays a crucial role in lipid metabolism . This inhibition can lead to decreased plasma free fatty acid levels, making these compounds potential candidates for treating metabolic disorders like insulin resistance and dyslipidemia.
- Antimicrobial Activity : Research indicates that boronic acids can exhibit antimicrobial properties by disrupting bacterial cell wall synthesis or inhibiting key metabolic pathways. The specific activity of this compound against various pathogens remains an area of ongoing investigation.
Therapeutic Applications
The therapeutic potential of this compound spans several areas:
- Metabolic Disorders : Due to its ability to inhibit HSL, this compound may be beneficial in managing conditions associated with lipid metabolism disorders, including type 2 diabetes and metabolic syndrome .
- Cancer Treatment : Boronic acids have been explored for their role in cancer therapeutics, particularly due to their ability to interfere with proteasomal degradation pathways . This suggests potential applications in developing anti-cancer agents.
Case Studies and Research Findings
Recent studies have highlighted the biological activities of boronic acids:
- Sphingosine Kinase Inhibition : A study demonstrated that specific boronic acid derivatives could selectively inhibit sphingosine kinase 1 (SphK1), impacting cell survival and migration pathways . While this compound was not the primary focus, its structural analogs show promise in this area.
- Antimicrobial Efficacy : Research into a library of oxazolidinones indicated that certain boronic acid derivatives exhibited significant activity against Gram-negative bacteria by overcoming efflux mechanisms . This suggests that this compound could be evaluated for similar properties.
Data Table: Biological Activities of Boronic Acids
Q & A
Basic Question: What are the standard synthetic routes for aromatic boronic acids like (5-Butoxy-2,4-dichlorophenyl)boronic acid, and what purification challenges arise due to their reactivity?
Methodological Answer:
Aromatic boronic acids are typically synthesized via Miyaura borylation, where halogenated precursors (e.g., aryl bromides or chlorides) react with bis(pinacolato)diboron under palladium catalysis . For derivatives with bulky substituents (e.g., butoxy and dichloro groups), steric hindrance may necessitate optimized ligand systems (e.g., SPhos or XPhos) to improve yield. Post-synthesis, boronic acids are prone to trimerization into boroxines, complicating purification. To mitigate this, intermediates are often isolated as pinacol esters, which are hydrolyzed under mild acidic conditions . Reverse-phase HPLC or recrystallization in non-polar solvents (e.g., hexane/ethyl acetate) can resolve dehydration byproducts.
Q. Advanced Question: How can stereochemical control be achieved during the synthesis of boronic acid derivatives with multiple halogen substituents?
Methodological Answer: Stereochemical control in polyhalogenated boronic acids requires careful selection of protecting groups and reaction conditions. For example, orthogonal protection of reactive sites (e.g., using silyl ethers for alcohols) prevents undesired cross-coupling during borylation . Computational modeling (DFT calculations) can predict regioselectivity in halogenated precursors, guiding the choice of catalysts. For instance, bulky ligands like DavePhos enhance selectivity in meta-substituted aryl halides . Post-synthetic characterization via B NMR and X-ray crystallography validates structural integrity .
Basic Question: What analytical techniques are most effective for characterizing boronic acid trimerization and ensuring sample purity?
Methodological Answer:
Mass spectrometry (MS) with matrix-assisted laser desorption/ionization (MALDI) is preferred for detecting boroxine formation. Derivatization with diols (e.g., pinacol) stabilizes boronic acids as esters, eliminating trimerization artifacts during analysis . B NMR spectroscopy is critical for identifying boron environments: free boronic acids resonate at δ ~30 ppm, while boroxines appear at δ ~18 ppm . Purity assessment via HPLC-UV (C18 columns, acetonitrile/water gradients) resolves residual precursors and dehydration products .
Q. Advanced Question: How can LC-MS/MS be optimized to quantify trace boronic acid impurities in drug substances?
Methodological Answer: Triple quadrupole LC-MS/MS in multiple reaction monitoring (MRM) mode offers high sensitivity for sub-ppm impurity detection. For underivatized boronic acids, electrospray ionization (ESI) in negative ion mode enhances detection limits. Mobile phases with 0.1% formic acid improve ionization efficiency, while column temperatures ≤25°C minimize on-column degradation . Method validation per ICH guidelines requires spike-recovery experiments (80–120% recovery) and robustness testing against pH (±0.2) and flow rate (±10%) variations .
Basic Question: What biological targets are most relevant for boronic acid-based drug discovery, and how are activity assays designed?
Methodological Answer:
Boronic acids are leveraged as proteasome inhibitors (e.g., targeting the β5 subunit), thrombin inhibitors, and tubulin polymerization disruptors . Assays include:
- Proteasome inhibition: Fluorescent substrates (e.g., Suc-LLVY-AMC) quantify chymotrypsin-like activity in cell lysates .
- Tubulin polymerization: Turbidity measurements at 350 nm track microtubule assembly in vitro .
- Cellular uptake: Flow cytometry with boron-conjugated fluorophores (e.g., BODIPY) evaluates membrane permeability .
Q. Advanced Question: How do researchers reconcile conflicting data between in vitro enzyme inhibition and cellular efficacy for boronic acid drugs?
Methodological Answer: Discrepancies often arise from off-target binding or poor pharmacokinetics. To address this:
Competitive ABPP (Activity-Based Protein Profiling): Identifies off-target interactions using broad-spectrum probes .
Metabolic stability assays: Liver microsome incubations (human/rat) assess cytochrome P450-mediated degradation .
Tissue distribution studies: Radiolabeled F-boronic acids track biodistribution via PET imaging .
Basic Question: How do boronic acids interact with diols in aqueous environments, and how is binding kinetics measured?
Methodological Answer:
Boronic acids form reversible esters with 1,2- or 1,3-diols (e.g., sugars) via nucleophilic attack on the boron atom. Binding affinity () is quantified via isothermal titration calorimetry (ITC) or fluorescence displacement assays (e.g., Alizarin Red S competition) . Stopped-flow kinetics reveal values (10–10 Ms) for rapid equilibration (<10 s) .
Q. Advanced Question: How can photo-responsive boronic acids enable spatiotemporal control of diol binding in biological systems?
Methodological Answer: Azobenzene-boronic acid conjugates undergo E→Z isomerization under visible light, modulating diol affinity. For example, 2,6-dimethoxy azobenzene derivatives exhibit a 20-fold enhancement upon irradiation (λ = 450 nm), enabling "catch-and-release" of glycoproteins in hydrogels . Red-light activation minimizes phototoxicity, making these systems suitable for in vivo glucose monitoring .
Basic Question: What role do boronic acids play in materials science, particularly in flame retardancy?
Methodological Answer:
Aromatic boronic acids decompose endothermically under heat, releasing boron oxides that form protective glassy layers on polymers. Thermogravimetric analysis (TGA) under nitrogen (10°C/min) quantifies char residue (>30% at 700°C for meta-substituted derivatives) . Halogen-free formulations combine boronic acids with phosphazenes to meet UL94 V-0 ratings .
Q. Advanced Question: How does the substitution pattern on boronic acids influence their thermal stability and flame-retardant efficiency?
Methodological Answer: Electron-withdrawing groups (e.g., -Cl, -CF) lower decomposition onset temperatures but enhance char yield. Ortho-substitution increases steric hindrance, delaying boroxine formation and improving thermal stability. Computational models (ReaxFF MD simulations) predict degradation pathways, guiding the design of boronic acid-polycarbonate composites .
Basic Question: How are boronic acids utilized in drug delivery systems to enhance cellular uptake?
Methodological Answer:
Boronic acids bind cell-surface glycoproteins (e.g., GLUT1), facilitating receptor-mediated endocytosis. Liposomes functionalized with phenylboronic acid (PBA) show 5-fold higher uptake in cancer cells vs. free drugs . pH-responsive micelles (boronate ester crosslinks) release payloads in acidic tumor microenvironments .
Q. Advanced Question: What strategies minimize off-target binding of boronic acid-drug conjugates in vivo?
Methodological Answer: Dual-targeting systems combine boronic acids with peptide ligands (e.g., RGD for integrin-rich tumors) . Pre-blocking serum proteins (e.g., albumin) with free fructose reduces nonspecific interactions . PET imaging with F-labeled analogs validates targeting specificity .
Basic Question: What contradictions exist in the literature regarding boronic acid-diol binding selectivity?
Methodological Answer:
While boronic acids preferentially bind fructose ( ~10 M) over glucose ( ~10 M), non-specific interactions with arginine-rich proteins (e.g., avidin) can mask selectivity. SPR studies show secondary electrostatic interactions account for >40% of avidin binding to AECPBA surfaces .
Q. Advanced Question: How can researchers mitigate false positives in boronic acid-based glycoprotein sensors?
Methodological Answer: Coating surfaces with zwitterionic polymers (e.g., polySBMA) reduces nonspecific protein adsorption. Buffer optimization (e.g., 100 mM HEPES, pH 7.4) weakens ionic interactions while preserving boronate-diol binding . Competitive elution with sorbitol (500 mM) confirms specificity .
Basic Question: What advances enable boronic acids to act as photoresponsive switches in dynamic covalent chemistry?
Methodological Answer:
Azobenzene-boronic acid hybrids undergo reversible E/Z isomerization under visible light, altering diol binding affinity. For example, Z-isomers of 2,6-dimethoxy derivatives bind fructose 20-fold tighter than E-isomers, enabling light-controlled hydrogel stiffening .
Q. Advanced Question: How are these photoresponsive systems applied in real-time biosensing?
Methodological Answer: Conjugation to PEG-star polymers creates light-tunable hydrogels for continuous glucose monitoring. Red light (λ = 630 nm) shifts equilibrium toward high-affinity Z-isomers, correlating with fluorescence intensity changes (ΔF/F > 50%) .
Eigenschaften
IUPAC Name |
(5-butoxy-2,4-dichlorophenyl)boronic acid | |
---|---|---|
Source | PubChem | |
URL | https://pubchem.ncbi.nlm.nih.gov | |
Description | Data deposited in or computed by PubChem | |
InChI |
InChI=1S/C10H13BCl2O3/c1-2-3-4-16-10-5-7(11(14)15)8(12)6-9(10)13/h5-6,14-15H,2-4H2,1H3 | |
Source | PubChem | |
URL | https://pubchem.ncbi.nlm.nih.gov | |
Description | Data deposited in or computed by PubChem | |
InChI Key |
UNUSRHVSANUKKR-UHFFFAOYSA-N | |
Source | PubChem | |
URL | https://pubchem.ncbi.nlm.nih.gov | |
Description | Data deposited in or computed by PubChem | |
Canonical SMILES |
B(C1=CC(=C(C=C1Cl)Cl)OCCCC)(O)O | |
Source | PubChem | |
URL | https://pubchem.ncbi.nlm.nih.gov | |
Description | Data deposited in or computed by PubChem | |
Molecular Formula |
C10H13BCl2O3 | |
Source | PubChem | |
URL | https://pubchem.ncbi.nlm.nih.gov | |
Description | Data deposited in or computed by PubChem | |
DSSTOX Substance ID |
DTXSID30681689 | |
Record name | (5-Butoxy-2,4-dichlorophenyl)boronic acid | |
Source | EPA DSSTox | |
URL | https://comptox.epa.gov/dashboard/DTXSID30681689 | |
Description | DSSTox provides a high quality public chemistry resource for supporting improved predictive toxicology. | |
Molecular Weight |
262.92 g/mol | |
Source | PubChem | |
URL | https://pubchem.ncbi.nlm.nih.gov | |
Description | Data deposited in or computed by PubChem | |
CAS No. |
1256354-88-7 | |
Record name | (5-Butoxy-2,4-dichlorophenyl)boronic acid | |
Source | EPA DSSTox | |
URL | https://comptox.epa.gov/dashboard/DTXSID30681689 | |
Description | DSSTox provides a high quality public chemistry resource for supporting improved predictive toxicology. | |
Haftungsausschluss und Informationen zu In-Vitro-Forschungsprodukten
Bitte beachten Sie, dass alle Artikel und Produktinformationen, die auf BenchChem präsentiert werden, ausschließlich zu Informationszwecken bestimmt sind. Die auf BenchChem zum Kauf angebotenen Produkte sind speziell für In-vitro-Studien konzipiert, die außerhalb lebender Organismen durchgeführt werden. In-vitro-Studien, abgeleitet von dem lateinischen Begriff "in Glas", beinhalten Experimente, die in kontrollierten Laborumgebungen unter Verwendung von Zellen oder Geweben durchgeführt werden. Es ist wichtig zu beachten, dass diese Produkte nicht als Arzneimittel oder Medikamente eingestuft sind und keine Zulassung der FDA für die Vorbeugung, Behandlung oder Heilung von medizinischen Zuständen, Beschwerden oder Krankheiten erhalten haben. Wir müssen betonen, dass jede Form der körperlichen Einführung dieser Produkte in Menschen oder Tiere gesetzlich strikt untersagt ist. Es ist unerlässlich, sich an diese Richtlinien zu halten, um die Einhaltung rechtlicher und ethischer Standards in Forschung und Experiment zu gewährleisten.