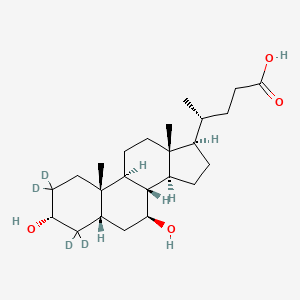
Ursodeoxycholic-2,2,4,4-D4 acid
Übersicht
Beschreibung
Ursodeoxycholsäure-d4 ist eine deuterierte Form der Ursodeoxycholsäure, einer sekundären Gallensäure, die durch Epimerisierung von Chenodesoxycholsäure gebildet wird. Ursodeoxycholsäure ist eine natürlich vorkommende Gallensäure, die einen geringen Anteil am menschlichen Gallensäurenpool ausmacht. Sie wird seit Jahrzehnten zur Behandlung von Lebererkrankungen eingesetzt und ist für ihre Hydrophilie und geringere Toxizität im Vergleich zu anderen Gallensäuren bekannt .
Wissenschaftliche Forschungsanwendungen
Ursodeoxycholsäure-d4 wird als interner Standard für die Quantifizierung von Ursodeoxycholsäure mittels Gaschromatographie oder Flüssigchromatographie-Massenspektrometrie verwendet. Sie wird auch zur Untersuchung des Gallensäurenstoffwechsels, der Krankheitsverknüpfung und der Biomarker-Bewertung eingesetzt .
In der Medizin wird Ursodeoxycholsäure zur Behandlung von primärer biliärer Zirrhose, Gallensteinen und anderen Lebererkrankungen eingesetzt. Es wurde gezeigt, dass sie die Apoptose hemmt, die durch Desoxycholsäure oder Ethanol in primären Rattenhepatocyten induziert wird .
Wirkmechanismus
Ursodeoxycholsäure entfaltet ihre Wirkung über mehrere Mechanismen:
Schutz von Cholangiozyten: Sie schützt Cholangiozyten vor der Zytotoxizität hydrophober Gallensäuren, indem sie die Zusammensetzung von gemischten Phospholipid-reichen Mizellen moduliert und die Gallensäurenzytotoxizität reduziert.
Stimulation der hepatobiliären Sekretion: Sie stimuliert die hepatobiliäre Sekretion über Calcium-abhängige und Protein-Kinase-C-alpha-abhängige Mechanismen, was zur Insertion von Transportermolekülen in die kanalikuläre Membran von Hepatozyten führt.
Hemmung der Apoptose: Sie hemmt die durch Gallensäuren induzierte Apoptose, indem sie die Bildung von mitochondrialen Poren und die Membranrekrutierung von Todesrezeptoren verhindert.
Wirkmechanismus
Target of Action
Ursodeoxycholic-2,2,4,4-D4 acid, a deuterium-labeled form of Ursodeoxycholic acid , primarily targets G-protein coupled bile acid receptor 5 (TGR5, GPCR19) and the farnesoid X receptor (FXR) . These receptors play a crucial role in maintaining the integrity of the intestinal barrier and are essential for lipid metabolism .
Mode of Action
This compound acts as a signaling molecule, exerting its effects by interacting with its targets . It binds to the TGR5 and FXR receptors, triggering a series of biochemical reactions that regulate lipid metabolism and maintain the integrity of the intestinal barrier .
Biochemical Pathways
The interaction of this compound with TGR5 and FXR receptors influences several biochemical pathways. These pathways primarily involve the regulation of lipid metabolism and the maintenance of intestinal barrier integrity . The exact downstream effects of these pathways are complex and depend on various factors, including the specific cellular context and the presence of other signaling molecules.
Pharmacokinetics
It’s known that the incorporation of deuterium into drug molecules can potentially affect their pharmacokinetic and metabolic profiles
Result of Action
The interaction of this compound with its targets leads to a variety of molecular and cellular effects. It plays a key role in regulating lipid metabolism and maintaining the integrity of the intestinal barrier . Additionally, it has been found to reduce ACE2 expression, which could potentially be beneficial for reducing SARS-CoV-2 infection .
Safety and Hazards
Zukünftige Richtungen
Biochemische Analyse
Biochemical Properties
Ursodeoxycholic-2,2,4,4-D4 acid interacts with various enzymes, proteins, and other biomolecules. It acts as a signaling molecule, exerting its effects by interacting with bile acid activated receptors, including G-protein coupled bile acid receptor 5 (TGR5, GPCR19) and the farnesoid X receptor (FXR) .
Cellular Effects
This compound influences cell function by impacting cell signaling pathways, gene expression, and cellular metabolism . It also reduces ACE2 expression, which is beneficial for reducing SARS-CoV-2 infection .
Molecular Mechanism
At the molecular level, this compound exerts its effects through binding interactions with biomolecules, enzyme inhibition or activation, and changes in gene expression .
Metabolic Pathways
This compound is involved in various metabolic pathways. It interacts with enzymes or cofactors and can affect metabolic flux or metabolite levels .
Vorbereitungsmethoden
Synthesewege und Reaktionsbedingungen
Ursodeoxycholsäure-d4 kann durch Epimerisierung von Chenodesoxycholsäure unter Verwendung von 7α- und 7β-Hydroxysteroid-Dehydrogenasen synthetisiert werden. Diese Enzyme sind wichtige Biokatalysatoren für den Biotransformationsprozess. Die Enzyme werden heterolog in Escherichia coli BL21 exprimiert, und die Wirkung der enzymatischen Synthese wird mittels Massenspektrometrie, IR-Spektrum und NMR untersucht .
Ein weiteres Verfahren beinhaltet die Elektroreduktion von 7-Keton-Lithocholsäure. Dieser Prozess verwendet Dimethylsulfoxid, Dimethylformamid und N-Methyl-2-Pyrrolidon als Stereoselektivitätsadditive während der Elektroreduktion. Das Elektrolysesystem erreicht eine hohe Umwandlungsrate und Ausbeute an Ursodeoxycholsäure .
Industrielle Produktionsverfahren
Die großtechnische Herstellung von Ursodeoxycholsäure aus Chenodesoxycholsäure erfolgt unter Verwendung von 7α- und 7β-Hydroxysteroid-Dehydrogenasen. Diese Enzyme werden in einem 500-L-Fermenter produziert, wodurch die industrielle Anwendung des Verfahrens ermöglicht wird. Das Produktionsverfahren zeichnet sich durch hohe Effizienz und Umweltfreundlichkeit im Vergleich zu herkömmlichen chemischen Syntheseverfahren aus .
Analyse Chemischer Reaktionen
Arten von Reaktionen
Ursodeoxycholsäure-d4 durchläuft verschiedene chemische Reaktionen, darunter:
Oxidation: Sie kann zu 7-Keton-Derivaten oxidiert werden.
Reduktion: Elektroreduktion von 7-Keton-Lithocholsäure zu Ursodeoxycholsäure.
Substitution: Sie kann Substitutionsreaktionen mit verschiedenen Reagenzien eingehen.
Häufige Reagenzien und Bedingungen
Oxidation: Häufige Oxidationsmittel sind Kaliumpermanganat und Chromtrioxid.
Reduktion: Bei der Elektroreduktion werden Dimethylsulfoxid, Dimethylformamid und N-Methyl-2-Pyrrolidon als Additive verwendet.
Substitution: Reagenzien wie Natriumhydroxid und Salzsäure werden häufig eingesetzt.
Wichtigste gebildete Produkte
Oxidation: 7-Keton-Derivate.
Reduktion: Ursodeoxycholsäure.
Substitution: Verschiedene substituierte Gallensäuren.
Vergleich Mit ähnlichen Verbindungen
Ähnliche Verbindungen
Chenodesoxycholsäure: Eine primäre Gallensäure, die durch Epimerisierung zu Ursodeoxycholsäure umgewandelt wird.
Lithocholsäure: Ein Metabolit der Ursodeoxycholsäure in menschlichen Lebermikrosomen.
Taurocholsäure: Eine weitere Gallensäure, die weniger hydrophil ist als Ursodeoxycholsäure
Einzigartigkeit
Ursodeoxycholsäure ist einzigartig aufgrund ihrer Hydrophilie und geringeren Toxizität im Vergleich zu anderen Gallensäuren. Sie ist weniger toxisch als Cholsäure oder Chenodesoxycholsäure und wird seit Jahrzehnten zur Behandlung von Lebererkrankungen eingesetzt .
Eigenschaften
IUPAC Name |
(4R)-4-[(3R,5S,7S,8R,9S,10S,13R,14S,17R)-2,2,4,4-tetradeuterio-3,7-dihydroxy-10,13-dimethyl-3,5,6,7,8,9,11,12,14,15,16,17-dodecahydro-1H-cyclopenta[a]phenanthren-17-yl]pentanoic acid | |
---|---|---|
Source | PubChem | |
URL | https://pubchem.ncbi.nlm.nih.gov | |
Description | Data deposited in or computed by PubChem | |
InChI |
InChI=1S/C24H40O4/c1-14(4-7-21(27)28)17-5-6-18-22-19(9-11-24(17,18)3)23(2)10-8-16(25)12-15(23)13-20(22)26/h14-20,22,25-26H,4-13H2,1-3H3,(H,27,28)/t14-,15+,16-,17-,18+,19+,20+,22+,23+,24-/m1/s1/i8D2,12D2 | |
Source | PubChem | |
URL | https://pubchem.ncbi.nlm.nih.gov | |
Description | Data deposited in or computed by PubChem | |
InChI Key |
RUDATBOHQWOJDD-QGCKUCIHSA-N | |
Source | PubChem | |
URL | https://pubchem.ncbi.nlm.nih.gov | |
Description | Data deposited in or computed by PubChem | |
Canonical SMILES |
CC(CCC(=O)O)C1CCC2C1(CCC3C2C(CC4C3(CCC(C4)O)C)O)C | |
Source | PubChem | |
URL | https://pubchem.ncbi.nlm.nih.gov | |
Description | Data deposited in or computed by PubChem | |
Isomeric SMILES |
[2H]C1(C[C@@]2([C@H]3CC[C@]4([C@H]([C@@H]3[C@H](C[C@@H]2C([C@@H]1O)([2H])[2H])O)CC[C@@H]4[C@H](C)CCC(=O)O)C)C)[2H] | |
Source | PubChem | |
URL | https://pubchem.ncbi.nlm.nih.gov | |
Description | Data deposited in or computed by PubChem | |
Molecular Formula |
C24H40O4 | |
Source | PubChem | |
URL | https://pubchem.ncbi.nlm.nih.gov | |
Description | Data deposited in or computed by PubChem | |
Molecular Weight |
396.6 g/mol | |
Source | PubChem | |
URL | https://pubchem.ncbi.nlm.nih.gov | |
Description | Data deposited in or computed by PubChem | |
CAS No. |
347841-46-7 | |
Record name | (3α,5β,7β)-3,7-Dihydroxycholan-24-oic-2,2,4,4-d4 acid | |
Source | CAS Common Chemistry | |
URL | https://commonchemistry.cas.org/detail?cas_rn=347841-46-7 | |
Description | CAS Common Chemistry is an open community resource for accessing chemical information. Nearly 500,000 chemical substances from CAS REGISTRY cover areas of community interest, including common and frequently regulated chemicals, and those relevant to high school and undergraduate chemistry classes. This chemical information, curated by our expert scientists, is provided in alignment with our mission as a division of the American Chemical Society. | |
Explanation | The data from CAS Common Chemistry is provided under a CC-BY-NC 4.0 license, unless otherwise stated. | |
Haftungsausschluss und Informationen zu In-Vitro-Forschungsprodukten
Bitte beachten Sie, dass alle Artikel und Produktinformationen, die auf BenchChem präsentiert werden, ausschließlich zu Informationszwecken bestimmt sind. Die auf BenchChem zum Kauf angebotenen Produkte sind speziell für In-vitro-Studien konzipiert, die außerhalb lebender Organismen durchgeführt werden. In-vitro-Studien, abgeleitet von dem lateinischen Begriff "in Glas", beinhalten Experimente, die in kontrollierten Laborumgebungen unter Verwendung von Zellen oder Geweben durchgeführt werden. Es ist wichtig zu beachten, dass diese Produkte nicht als Arzneimittel oder Medikamente eingestuft sind und keine Zulassung der FDA für die Vorbeugung, Behandlung oder Heilung von medizinischen Zuständen, Beschwerden oder Krankheiten erhalten haben. Wir müssen betonen, dass jede Form der körperlichen Einführung dieser Produkte in Menschen oder Tiere gesetzlich strikt untersagt ist. Es ist unerlässlich, sich an diese Richtlinien zu halten, um die Einhaltung rechtlicher und ethischer Standards in Forschung und Experiment zu gewährleisten.