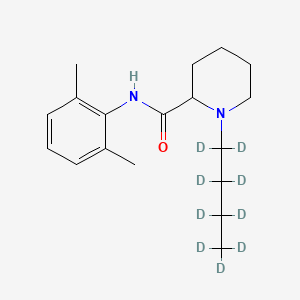
Bupivacaine-d9
- Klicken Sie auf QUICK INQUIRY, um ein Angebot von unserem Expertenteam zu erhalten.
- Mit qualitativ hochwertigen Produkten zu einem WETTBEWERBSFÄHIGEN Preis können Sie sich mehr auf Ihre Forschung konzentrieren.
Übersicht
Beschreibung
Bupivacaine-d9 is a potent local anesthetic from the amide group of local anesthetics . It is intended for use as an internal standard for the quantification of bupivacaine by GC- or LC-MS . Bupivacaine-d9 inhibits sodium currents in rat dorsal horn neurons in a concentration-dependent manner and inhibits synaptic transmission in rat sympathetic ganglia . It also blocks cardiac sodium channels in a use-dependent manner and inhibits respiration in cardiac cell mitochondria . Bupivacaine-d9 is used in regional anesthesia, epidural anesthesia, spinal anesthesia, and local infiltration .
Molecular Structure Analysis
Bupivacaine-d9 has a molecular formula of C18H19D9N2O and a molecular weight of 297.5 g/mol . It contains three structural components: an aromatic ring, a connecting group which is an amide, and an ionizable amine group .
Physical And Chemical Properties Analysis
Bupivacaine-d9 has a molecular formula of C18H19D9N2O and a molecular weight of 297.5 g/mol . The exact physical and chemical properties of Bupivacaine-d9 are not detailed in the search results.
Wissenschaftliche Forschungsanwendungen
Cancer Treatment and Management
Bupivacaine-d9: has been explored for its potential in cancer treatment, particularly in the context of the tumor microenvironment. Researchers have designed PEGylated lipid nanoparticles loaded with bupivacaine to target neurons within breast cancer tumors. This approach aims to suppress nerve-to-cancer cross-talk, which is known to promote cancer progression. The encapsulation of bupivacaine into nanoparticles has shown promise in reducing systemic adverse effects and inhibiting tumor growth and metastatic dissemination .
Pain Management in Clinical Settings
In the field of pain management, Bupivacaine-d9 has been utilized in osmotically balanced, large unilamellar liposomes for sustained release. This method provides prolonged pain relief in in vivo rat models, simulating postoperative pain. The liposomes’ ability to release bupivacaine over an extended period has proven effective in managing acute postoperative pain, which is a crucial issue for clinicians .
Pharmacokinetics and Safety Studies
The pharmacokinetics and safety of liposomal bupivacaine, a long-acting formulation of bupivacaine, have been studied across various surgical procedures. Research has been conducted to assess these parameters specifically in the Chinese population, where such data had not been previously evaluated .
Wirkmechanismus
Target of Action
Bupivacaine-d9, like its parent compound Bupivacaine, primarily targets sodium channels on nerve cell membranes . These channels play a crucial role in the initiation and conduction of nerve impulses. By interacting with these channels, Bupivacaine-d9 can influence the transmission of signals in the nervous system .
Mode of Action
Bupivacaine-d9 acts by binding to sodium channels and inhibiting the influx of sodium ions . This action decreases the neuronal membrane’s permeability to sodium ions, resulting in inhibition of depolarization. Consequently, this blockade prevents the propagation of nerve impulses, leading to a loss of sensation in the targeted area .
Biochemical Pathways
Bupivacaine-d9 affects several biochemical pathways. For instance, it has been found to modulate apoptosis and ferroptosis in bladder cancer via the phosphatidylinositol 3-kinase (PI3K)/AKT pathway . Additionally, it has been reported to regulate the BDNF-TrkB/proBDNF-p75NTR pathway, which is involved in neuronal maturation and oxidative stress .
Pharmacokinetics
Bupivacaine-d9, like Bupivacaine, is extensively and rapidly metabolized in the liver, followed by excretion via urine . Less than 10% of the dose is excreted as the parent compound, Bupivacaine . The pharmacokinetic properties of Bupivacaine-d9 are expected to be similar to those of Bupivacaine.
Result of Action
The primary result of Bupivacaine-d9 action is the temporary numbness or loss of sensation in specific areas of the body . On a molecular level, it has been found to induce apoptosis and ferroptosis in bladder cancer cells by inhibiting the PI3K/Akt signaling pathway . In non-small cell lung cancer, Bupivacaine-d9 has been reported to inhibit progression by inducing autophagy through the Akt/mTOR signaling pathway .
Action Environment
Environmental factors can influence the action, efficacy, and stability of Bupivacaine-d9. For instance, the presence of other drugs, the patient’s metabolic state, and the specific physiological environment can impact the drug’s effectiveness . .
Safety and Hazards
Bupivacaine-d9 is harmful if swallowed, inhaled, or in contact with skin . It may cause respiratory irritation and skin irritation . It is advised to wear personal protective equipment/face protection, avoid dust formation, and not to get in eyes, on skin, or on clothing . It should be used only under a chemical fume hood .
Eigenschaften
IUPAC Name |
N-(2,6-dimethylphenyl)-1-(1,1,2,2,3,3,4,4,4-nonadeuteriobutyl)piperidine-2-carboxamide |
Source
|
---|---|---|
Source | PubChem | |
URL | https://pubchem.ncbi.nlm.nih.gov | |
Description | Data deposited in or computed by PubChem | |
InChI |
InChI=1S/C18H28N2O/c1-4-5-12-20-13-7-6-11-16(20)18(21)19-17-14(2)9-8-10-15(17)3/h8-10,16H,4-7,11-13H2,1-3H3,(H,19,21)/i1D3,4D2,5D2,12D2 |
Source
|
Source | PubChem | |
URL | https://pubchem.ncbi.nlm.nih.gov | |
Description | Data deposited in or computed by PubChem | |
InChI Key |
LEBVLXFERQHONN-JOJYFGIVSA-N |
Source
|
Source | PubChem | |
URL | https://pubchem.ncbi.nlm.nih.gov | |
Description | Data deposited in or computed by PubChem | |
Canonical SMILES |
CCCCN1CCCCC1C(=O)NC2=C(C=CC=C2C)C |
Source
|
Source | PubChem | |
URL | https://pubchem.ncbi.nlm.nih.gov | |
Description | Data deposited in or computed by PubChem | |
Isomeric SMILES |
[2H]C([2H])([2H])C([2H])([2H])C([2H])([2H])C([2H])([2H])N1CCCCC1C(=O)NC2=C(C=CC=C2C)C |
Source
|
Source | PubChem | |
URL | https://pubchem.ncbi.nlm.nih.gov | |
Description | Data deposited in or computed by PubChem | |
Molecular Formula |
C18H28N2O |
Source
|
Source | PubChem | |
URL | https://pubchem.ncbi.nlm.nih.gov | |
Description | Data deposited in or computed by PubChem | |
Molecular Weight |
297.5 g/mol |
Source
|
Source | PubChem | |
URL | https://pubchem.ncbi.nlm.nih.gov | |
Description | Data deposited in or computed by PubChem | |
Q & A
Q1: Why is Bupivacaine-d9 chosen as an internal standard for Bupivacaine in this study?
A1: Bupivacaine-d9 is a deuterated form of Bupivacaine, meaning it possesses the same chemical structure with the exception of nine hydrogen atoms substituted by deuterium atoms. This isotopic substitution results in similar chemical behavior to Bupivacaine during sample preparation and analysis, but with a distinguishable mass difference detectable by mass spectrometry [].
Q2: How does the use of Bupivacaine-d9 contribute to the validation of the UPLC-MS/MS method?
A2: In the presented research [], the incorporation of Bupivacaine-d9 is essential for method validation, specifically contributing to:
Haftungsausschluss und Informationen zu In-Vitro-Forschungsprodukten
Bitte beachten Sie, dass alle Artikel und Produktinformationen, die auf BenchChem präsentiert werden, ausschließlich zu Informationszwecken bestimmt sind. Die auf BenchChem zum Kauf angebotenen Produkte sind speziell für In-vitro-Studien konzipiert, die außerhalb lebender Organismen durchgeführt werden. In-vitro-Studien, abgeleitet von dem lateinischen Begriff "in Glas", beinhalten Experimente, die in kontrollierten Laborumgebungen unter Verwendung von Zellen oder Geweben durchgeführt werden. Es ist wichtig zu beachten, dass diese Produkte nicht als Arzneimittel oder Medikamente eingestuft sind und keine Zulassung der FDA für die Vorbeugung, Behandlung oder Heilung von medizinischen Zuständen, Beschwerden oder Krankheiten erhalten haben. Wir müssen betonen, dass jede Form der körperlichen Einführung dieser Produkte in Menschen oder Tiere gesetzlich strikt untersagt ist. Es ist unerlässlich, sich an diese Richtlinien zu halten, um die Einhaltung rechtlicher und ethischer Standards in Forschung und Experiment zu gewährleisten.