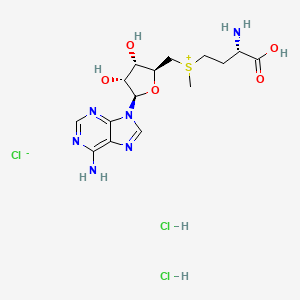
S-(5'-Adenosyl)-L-methionine chloride dihydrochloride
Übersicht
Beschreibung
Chemical Identity and Properties S-(5'-Adenosyl)-L-methionine chloride dihydrochloride (CAS: 86867-01-8) is a biologically active sulfonium compound formed by the enzymatic conjugation of methionine and adenosine triphosphate (ATP). It serves as a universal methyl donor in numerous biochemical reactions, including DNA/RNA/protein methylation, polyamine synthesis, and transsulfuration pathways .
Vorbereitungsmethoden
Synthetic Routes and Reaction Conditions: The synthesis of S-(5’-Adenosyl)-L-methionine chloride dihydrochloride typically involves the reaction of methionine with adenosine triphosphate (ATP) in the presence of methionine adenosyltransferase. The reaction conditions often require a buffered aqueous solution at a pH of around 7.5 to 8.0 and a temperature of approximately 37°C. The product is then purified through various chromatographic techniques to obtain the desired compound in its chloride dihydrochloride form.
Industrial Production Methods: Industrial production of S-(5’-Adenosyl)-L-methionine chloride dihydrochloride follows similar synthetic routes but on a larger scale. The process involves the use of bioreactors to facilitate the enzymatic reaction, followed by large-scale purification methods such as ion-exchange chromatography and crystallization to achieve high purity and yield.
Analyse Chemischer Reaktionen
Types of Reactions: S-(5’-Adenosyl)-L-methionine chloride dihydrochloride undergoes various chemical reactions, including:
Methylation: It acts as a methyl donor in transmethylation reactions.
Oxidation: It can be oxidized to form S-adenosyl-L-homocysteine.
Hydrolysis: It can be hydrolyzed to yield methionine and adenosine.
Common Reagents and Conditions:
Methylation: Requires methyltransferase enzymes and substrates such as DNA, RNA, or proteins.
Oxidation: Involves oxidizing agents like hydrogen peroxide or enzymatic oxidation.
Hydrolysis: Typically occurs under acidic or enzymatic conditions.
Major Products Formed:
Methylation: Produces methylated biomolecules and S-adenosyl-L-homocysteine.
Oxidation: Yields S-adenosyl-L-homocysteine.
Hydrolysis: Results in methionine and adenosine.
Wissenschaftliche Forschungsanwendungen
S-(5’-Adenosyl)-L-methionine chloride dihydrochloride is widely used in scientific research due to its role in methylation processes. Its applications include:
Chemistry: Used as a methyl donor in synthetic organic chemistry.
Biology: Involved in the study of gene expression and epigenetic modifications.
Medicine: Investigated for its potential therapeutic effects in treating liver diseases, depression, and osteoarthritis.
Industry: Utilized in the production of pharmaceuticals and as a dietary supplement.
Wirkmechanismus
The compound exerts its effects primarily through its role as a methyl donor in transmethylation reactions. It transfers a methyl group to various substrates, including DNA, RNA, proteins, and lipids, thereby influencing gene expression, protein function, and cellular metabolism. The molecular targets include methyltransferase enzymes, which facilitate the transfer of the methyl group from S-(5’-Adenosyl)-L-methionine chloride dihydrochloride to the substrate.
Vergleich Mit ähnlichen Verbindungen
Key Characteristics
- Molecular Formula : C₁₅H₂₃ClN₆O₅S·2HCl
- Molecular Weight : 507.82 g/mol
- Purity : ≥75% (HPLC)
- Storage : Requires storage at -20°C to maintain stability .
- Applications : Used in enzyme assays (e.g., methyltransferase activity), cancer stem cell research, and metabolic studies .
Mechanistic Role The compound donates methyl groups via its sulfonium center, facilitating reactions catalyzed by methyltransferases. For example, it participates in the methylation of histones (e.g., NSD3SET domain interactions ), RNA/DNA sensors , and small molecules like phloridzin docosahexaenoate (PZ-DHA) .
A detailed functional and structural comparison with related compounds is provided below.
S-Adenosylhomocysteine (SAH)
Role : SAH is the demethylated byproduct of SAM-dependent reactions and acts as a competitive inhibitor of methyltransferases .
- Functional Contrast :
5-Azacytidine (5-Aza)
Role : A DNA hypomethylating agent that inhibits DNA methyltransferases by covalent trapping .
- Functional Contrast: SAM promotes methylation (e.g., CD44/CD24 promoter methylation in cancer stem cells), whereas 5-Aza reverses it, inducing differentiation . In apoptosis reversal studies, SAM-treated cells retained methylation-dependent stemness, while 5-Aza disrupted this phenotype .
Other S-Adenosyl Derivatives
Comparative Data Table
Enzymatic Studies
- SAM is critical in O-methyltransferase assays, such as the biosynthesis of 1,4-dimethoxybenzene in pumpkin flowers .
- In ITC (isothermal titration calorimetry) studies, SAM binds to NSD3SET domains with micromolar affinity, highlighting its role in histone methylation .
Cancer Research
- SAM (0.5 mM) enhanced methylation-dependent CD44/CD24 promoter activity in cancer stem cells, whereas 5-Aza reversed this effect .
- SAM supplementation in liver microsomes facilitated the phase II metabolism of PZ-DHA, producing trimethylated and glucuronidated metabolites .
Technical Considerations
Biologische Aktivität
S-(5'-Adenosyl)-L-methionine chloride dihydrochloride (commonly referred to as SAM) is a biologically active compound that plays a crucial role in various biochemical processes. It is primarily known for its function as a methyl donor in methylation reactions, impacting proteins, lipids, and nucleic acids. This article delves into the biological activity of SAM, highlighting its mechanisms, therapeutic applications, and relevant research findings.
SAM is synthesized from methionine and adenosine triphosphate (ATP) through the action of methionine adenosyl transferase. Its primary biological role involves facilitating the transfer of methyl groups, which is vital for:
- Gene Regulation : Methylation of DNA influences gene expression and stability.
- Protein Modification : SAM contributes to post-translational modifications of proteins, affecting their function and interactions.
- Lipid Metabolism : It participates in the methylation of phospholipids, impacting membrane fluidity and function.
Therapeutic Applications
SAM has been explored for various therapeutic applications, particularly in the context of:
- Osteoarthritis : SAM supplementation has shown promise in reducing pain and improving joint function in patients with osteoarthritis.
- Liver Health : It is utilized as a treatment for liver diseases, including alcoholic liver disease and non-alcoholic fatty liver disease.
- Depression : Some studies suggest that SAM may have antidepressant effects comparable to traditional medications.
1. Cancer Proliferation and Bioenergetics
A study investigated the effects of SAM on colorectal cancer cell lines (HCT116). The findings indicated that low concentrations of SAM enhanced cellular bioenergetics and proliferation by stimulating cystathionine-β-synthase (CBS) activity, which leads to increased hydrogen sulfide (H₂S) production. However, prolonged exposure resulted in reduced ATP levels and cell viability, suggesting a bell-shaped dose-response relationship .
2. Neuroprotective Effects
Research has demonstrated that SAM exhibits neuroprotective properties by inhibiting acetylcholinesterase (AChE), an enzyme associated with neurodegenerative diseases such as Alzheimer's. In vitro studies showed varying degrees of AChE inhibition by different SAM derivatives, indicating its potential as a therapeutic agent for cognitive disorders .
3. Antioxidant Activity
SAM has also been recognized for its antioxidant capabilities. It plays a role in maintaining redox balance within cells, thereby protecting against oxidative stress. This activity is particularly relevant in conditions such as liver injury, where oxidative damage is prevalent .
Data Table: Summary of Biological Activities
Q & A
Basic Research Questions
Q. How should S-(5'-Adenosyl)-L-methionine chloride dihydrochloride (SAM) be handled and stored to ensure stability in experimental settings?
SAM is highly hygroscopic and prone to degradation under suboptimal conditions. For short-term use (≤1 week), store lyophilized SAM at -20°C in a desiccated environment. For long-term storage, aliquot the compound into single-use vials under inert gas (e.g., argon) to minimize oxidative degradation. Avoid repeated freeze-thaw cycles, as they accelerate hydrolysis of the sulfonium ion, reducing methyl donor activity . Reconstitute SAM in acidic buffers (pH 2–3) to stabilize the sulfonium moiety, and use immediately in enzymatic assays .
Q. What methodological approaches are recommended for quantifying SAM in biological samples?
Competitive ELISA kits (e.g., CEG414Ge, MEG414Ge) are widely used for SAM quantification in serum, plasma, and cell lysates, with detection limits of 4.63–5.51 ng/mL and linear ranges of 12.35–1,000 ng/mL . For higher specificity, pair HPLC-MS/MS with stable isotope-labeled SAM (e.g., S-(5′-Adenosyl)-L-methionine-(S-methyl-13C) chloride) as an internal standard. Use C18 reversed-phase columns with mobile phases containing ion-pairing agents (e.g., heptafluorobutyric acid) to resolve SAM from structural analogs like S-adenosylhomocysteine (SAH) .
Q. How can researchers assess the purity of commercial SAM and account for impurities in experimental outcomes?
Commercial SAM typically has a purity of ≥75% (by HPLC), with impurities including SAH, methionine, and adenosine . To validate purity:
- Perform HPLC-UV analysis at 254 nm using a zwitterionic HILIC column (e.g., ZIC-cHILIC) with isocratic elution (20 mM ammonium acetate, pH 5.0).
- Compare retention times against certified reference standards (e.g., USP SAM disulfate tosylate) .
- Adjust SAM concentrations in experiments based on measured purity to ensure stoichiometric accuracy in enzymatic reactions .
Q. What is the role of SAM in methyltransferase assays, and how does its stoichiometry influence reaction kinetics?
SAM serves as the primary methyl donor in methyltransferase reactions. Its sulfonium ion donates a methyl group to substrates (e.g., DNA, proteins, or small molecules), yielding SAH as a byproduct. Key considerations:
- Maintain a SAM:substrate molar ratio ≥ 2:1 to avoid rate-limiting depletion of SAM.
- Include Mg²+ (1–5 mM) as a cofactor for optimal enzyme activity in assays like nsp14 methyltransferase studies .
- Monitor SAH accumulation via coupled enzymatic assays (e.g., SAH hydrolase-linked NADH depletion) to quantify reaction progress .
Advanced Research Questions
Q. How should enzymatic assays be designed to address SAM’s instability and non-specific binding in high-throughput screens?
- Optimize buffer conditions : Use low-pH buffers (pH 6.5–7.0) with 1–2 mM DTT to stabilize SAM and reduce disulfide formation. Avoid Tris-based buffers, which react with SAM’s sulfonium group .
- Prevent non-specific adsorption : Add 0.01% BSA or 0.1% Tween-20 to reaction mixtures to minimize SAM binding to plasticware.
- Include SAH hydrolase inhibitors (e.g., 3-deazaadenosine) in cell-based assays to block SAH recycling and stabilize SAM pools .
Q. How do salt forms of SAM (e.g., chloride vs. tosylate) affect experimental reproducibility across studies?
- Solubility differences : SAM chloride dihydrochloride is highly soluble in water (>50 mg/mL), whereas tosylate salts require organic co-solvents (e.g., 10% DMSO) .
- Enzymatic activity : Tosylate counterions may inhibit certain methyltransferases (e.g., histone lysine methyltransferases) due to aromatic sulfonate interactions. Use chloride salts for these applications .
- Standardization : When comparing data across studies, normalize SAM concentrations to molar equivalents of the active sulfonium ion, regardless of salt form .
Q. What strategies resolve contradictory data in SAM-dependent enzyme kinetics caused by batch-to-batch variability?
- Batch validation : Pre-test each SAM batch in a control reaction with a well-characterized enzyme (e.g., human PRMT5). Compare initial reaction rates to historical data .
- SAH contamination correction : Quantify SAH levels via HPLC-UV and subtract background activity in no-substrate controls .
- Cross-validate with structural analogs : Use SAM analogs (e.g, sinefungin) to confirm substrate specificity and rule out off-target effects .
Q. How do structural features of SAM influence its interactions with methyltransferase active sites?
- Sulfonium ion geometry : The (3S)-configuration of SAM’s sulfonium group ensures proper alignment with catalytic residues (e.g., Tyr121 in human SETD2). Mutagenesis studies show that (3R)-isomers exhibit >90% reduced activity .
- Water-mediated H-bond networks : In crystallographic studies, SAM’s adenine ring forms H-bonds with conserved aspartate residues (e.g., Asp173 in SARS-CoV-2 nsp14), stabilizing the methyl-donor complex. Disrupting these interactions (e.g., via D173A mutation) abolishes activity .
- Dynamic simulations : Use molecular dynamics (MD) to model SAM’s conformational flexibility in enzyme pockets. Key metrics include RMSD (<1.5 Å) and binding free energy (ΔG ≤ -8 kcal/mol) .
Eigenschaften
IUPAC Name |
[(3S)-3-amino-3-carboxypropyl]-[[(2S,3S,4R,5R)-5-(6-aminopurin-9-yl)-3,4-dihydroxyoxolan-2-yl]methyl]-methylsulfanium;chloride;dihydrochloride | |
---|---|---|
Source | PubChem | |
URL | https://pubchem.ncbi.nlm.nih.gov | |
Description | Data deposited in or computed by PubChem | |
InChI |
InChI=1S/C15H22N6O5S.3ClH/c1-27(3-2-7(16)15(24)25)4-8-10(22)11(23)14(26-8)21-6-20-9-12(17)18-5-19-13(9)21;;;/h5-8,10-11,14,22-23H,2-4,16H2,1H3,(H2-,17,18,19,24,25);3*1H/t7-,8+,10+,11+,14+,27?;;;/m0.../s1 | |
Source | PubChem | |
URL | https://pubchem.ncbi.nlm.nih.gov | |
Description | Data deposited in or computed by PubChem | |
InChI Key |
KBAFOJZCBYWKPU-XQVUROGGSA-N | |
Source | PubChem | |
URL | https://pubchem.ncbi.nlm.nih.gov | |
Description | Data deposited in or computed by PubChem | |
Canonical SMILES |
C[S+](CCC(C(=O)O)N)CC1C(C(C(O1)N2C=NC3=C(N=CN=C32)N)O)O.Cl.Cl.[Cl-] | |
Source | PubChem | |
URL | https://pubchem.ncbi.nlm.nih.gov | |
Description | Data deposited in or computed by PubChem | |
Isomeric SMILES |
C[S+](CC[C@@H](C(=O)O)N)C[C@@H]1[C@H]([C@H]([C@@H](O1)N2C=NC3=C(N=CN=C32)N)O)O.Cl.Cl.[Cl-] | |
Source | PubChem | |
URL | https://pubchem.ncbi.nlm.nih.gov | |
Description | Data deposited in or computed by PubChem | |
Molecular Formula |
C15H25Cl3N6O5S | |
Source | PubChem | |
URL | https://pubchem.ncbi.nlm.nih.gov | |
Description | Data deposited in or computed by PubChem | |
Molecular Weight |
507.8 g/mol | |
Source | PubChem | |
URL | https://pubchem.ncbi.nlm.nih.gov | |
Description | Data deposited in or computed by PubChem | |
Haftungsausschluss und Informationen zu In-Vitro-Forschungsprodukten
Bitte beachten Sie, dass alle Artikel und Produktinformationen, die auf BenchChem präsentiert werden, ausschließlich zu Informationszwecken bestimmt sind. Die auf BenchChem zum Kauf angebotenen Produkte sind speziell für In-vitro-Studien konzipiert, die außerhalb lebender Organismen durchgeführt werden. In-vitro-Studien, abgeleitet von dem lateinischen Begriff "in Glas", beinhalten Experimente, die in kontrollierten Laborumgebungen unter Verwendung von Zellen oder Geweben durchgeführt werden. Es ist wichtig zu beachten, dass diese Produkte nicht als Arzneimittel oder Medikamente eingestuft sind und keine Zulassung der FDA für die Vorbeugung, Behandlung oder Heilung von medizinischen Zuständen, Beschwerden oder Krankheiten erhalten haben. Wir müssen betonen, dass jede Form der körperlichen Einführung dieser Produkte in Menschen oder Tiere gesetzlich strikt untersagt ist. Es ist unerlässlich, sich an diese Richtlinien zu halten, um die Einhaltung rechtlicher und ethischer Standards in Forschung und Experiment zu gewährleisten.