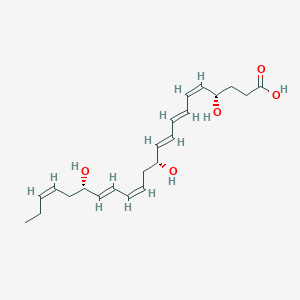
Resolvin D3
- Klicken Sie auf QUICK INQUIRY, um ein Angebot von unserem Expertenteam zu erhalten.
- Mit qualitativ hochwertigen Produkten zu einem WETTBEWERBSFÄHIGEN Preis können Sie sich mehr auf Ihre Forschung konzentrieren.
Übersicht
Beschreibung
Resolvin D3 is a specialized pro-resolving mediator derived from omega-3 fatty acids. It plays a crucial role in resolving inflammation and promoting tissue homeostasis. This compound is part of the resolvin family, which is known for its potent anti-inflammatory and pro-resolving properties.
Vorbereitungsmethoden
Synthesewege und Reaktionsbedingungen: Resolvin D3 kann durch eine Reihe von chemischen Reaktionen synthetisiert werden. Einer der wichtigsten Synthesewege beinhaltet die Suzuki-Miyaura-Kreuzkupplungsreaktion. Dieser Prozess umfasst die Kupplung einer Boranverbindung mit einem Iodolefin-Zwischenprodukt. Das Zwischenprodukt wird durch sequentielle Wittig-Reaktionen unter Verwendung von Phosphoniumsalzen und Aldehyden erhalten. Die stereogenen Zentren an bestimmten Positionen werden unter Verwendung der rutheniumkatalysierten asymmetrischen Transferhydrogenierung aufgebaut .
Industrielle Produktionsverfahren: Während detaillierte industrielle Produktionsverfahren nicht umfassend dokumentiert sind, kann der oben erwähnte Syntheseweg für industrielle Anwendungen hochskaliert werden. Die Verwendung effizienter Katalysatoren und optimierter Reaktionsbedingungen kann die Ausbeute und Reinheit von this compound verbessern.
Analyse Chemischer Reaktionen
Arten von Reaktionen: Resolvin D3 durchläuft verschiedene chemische Reaktionen, darunter Oxidation, Reduktion und Substitution. Diese Reaktionen sind essenziell für seine biologische Aktivität und Stabilität.
Häufige Reagenzien und Bedingungen:
Oxidation: Häufige Oxidationsmittel wie Wasserstoffperoxid oder molekularer Sauerstoff können verwendet werden.
Reduktion: Reduktionsmittel wie Natriumborhydrid oder Lithiumaluminiumhydrid werden eingesetzt.
Substitution: Nucleophile Substitutionsreaktionen können unter Verwendung geeigneter Nucleophile unter milden Bedingungen durchgeführt werden.
Hauptprodukte: Die Hauptprodukte, die aus diesen Reaktionen entstehen, umfassen verschiedene oxidierte oder reduzierte Derivate von this compound, die seine biologische Aktivität beibehalten oder verstärken.
Wissenschaftliche Forschungsanwendungen
Resolvin D3 hat eine große Bandbreite an Anwendungen in der wissenschaftlichen Forschung:
Chemie: Es wird als Modellverbindung verwendet, um die Synthese und Reaktivität von spezialisierten pro-auflösenden Mediatoren zu untersuchen.
Biologie: this compound wird für seine Rolle bei der Modulation von Immunantworten und der Auflösung von Entzündungen untersucht.
Industrie: this compound kann bei der Entwicklung von entzündungshemmenden Medikamenten und Nahrungsergänzungsmitteln verwendet werden.
5. Wirkmechanismus
This compound übt seine Wirkungen aus, indem es mit bestimmten molekularen Zielstrukturen und Signalwegen interagiert. Es bindet an G-Protein-gekoppelte Rezeptoren wie den N-Formylpeptid-Rezeptor 2 (ALX/FPR2), was zur Inhibition von pro-inflammatorischen Signalwegen führt. Diese Interaktion reduziert die Produktion von entzündungsfördernden Zytokinen und fördert die Auflösung von Entzündungen .
Ähnliche Verbindungen:
- Resolvin D1
- Resolvin E1
- Protectin D1
- Maresin 1
Vergleich: this compound ist einzigartig in seiner Fähigkeit, bestimmte Entzündungswege gezielt anzusprechen und zu modulieren. Im Vergleich zu anderen Resolvinen verfügt es über einzigartige strukturelle Merkmale und Stereochemie, die zu seinen spezifischen biologischen Aktivitäten beitragen. Zum Beispiel haben Resolvin D1 und Resolvin E1 ebenfalls entzündungshemmende Eigenschaften, aber sie interagieren mit verschiedenen Rezeptoren und Signalwegen .
This compound zeichnet sich durch seine starken Wirkungen auf die Reduzierung von Entzündungen und die Förderung der Gewebsreparatur aus, was es zu einem vielversprechenden Kandidaten für therapeutische Anwendungen macht.
Wirkmechanismus
Resolvin D3 exerts its effects by interacting with specific molecular targets and pathways. It binds to G-protein coupled receptors, such as the N-formyl peptide receptor 2 (ALX/FPR2), which leads to the inhibition of pro-inflammatory signaling pathways. This interaction reduces the production of inflammatory cytokines and promotes the resolution of inflammation .
Vergleich Mit ähnlichen Verbindungen
- Resolvin D1
- Resolvin E1
- Protectin D1
- Maresin 1
Comparison: Resolvin D3 is unique in its ability to specifically target and modulate certain inflammatory pathways. Compared to other resolvins, it has distinct structural features and stereochemistry that contribute to its specific biological activities. For example, Resolvin D1 and Resolvin E1 also have anti-inflammatory properties, but they interact with different receptors and pathways .
This compound stands out due to its potent effects on reducing inflammation and promoting tissue repair, making it a promising candidate for therapeutic applications.
Eigenschaften
IUPAC Name |
(4S,5Z,7E,9E,11R,13Z,15E,17S,19Z)-4,11,17-trihydroxydocosa-5,7,9,13,15,19-hexaenoic acid |
Source
|
---|---|---|
Source | PubChem | |
URL | https://pubchem.ncbi.nlm.nih.gov | |
Description | Data deposited in or computed by PubChem | |
InChI |
InChI=1S/C22H32O5/c1-2-3-7-12-19(23)14-10-6-11-15-20(24)13-8-4-5-9-16-21(25)17-18-22(26)27/h3-11,13-14,16,19-21,23-25H,2,12,15,17-18H2,1H3,(H,26,27)/b5-4+,7-3-,11-6-,13-8+,14-10+,16-9-/t19-,20-,21+/m0/s1 |
Source
|
Source | PubChem | |
URL | https://pubchem.ncbi.nlm.nih.gov | |
Description | Data deposited in or computed by PubChem | |
InChI Key |
QBTJOLCUKWLTIC-UZAFJXHNSA-N |
Source
|
Source | PubChem | |
URL | https://pubchem.ncbi.nlm.nih.gov | |
Description | Data deposited in or computed by PubChem | |
Canonical SMILES |
CCC=CCC(C=CC=CCC(C=CC=CC=CC(CCC(=O)O)O)O)O |
Source
|
Source | PubChem | |
URL | https://pubchem.ncbi.nlm.nih.gov | |
Description | Data deposited in or computed by PubChem | |
Isomeric SMILES |
CC/C=C\C[C@@H](/C=C/C=C\C[C@H](/C=C/C=C/C=C\[C@H](CCC(=O)O)O)O)O |
Source
|
Source | PubChem | |
URL | https://pubchem.ncbi.nlm.nih.gov | |
Description | Data deposited in or computed by PubChem | |
Molecular Formula |
C22H32O5 |
Source
|
Source | PubChem | |
URL | https://pubchem.ncbi.nlm.nih.gov | |
Description | Data deposited in or computed by PubChem | |
DSSTOX Substance ID |
DTXSID801345702 |
Source
|
Record name | Resolvin D3 | |
Source | EPA DSSTox | |
URL | https://comptox.epa.gov/dashboard/DTXSID801345702 | |
Description | DSSTox provides a high quality public chemistry resource for supporting improved predictive toxicology. | |
Molecular Weight |
376.5 g/mol |
Source
|
Source | PubChem | |
URL | https://pubchem.ncbi.nlm.nih.gov | |
Description | Data deposited in or computed by PubChem | |
CAS No. |
916888-47-6 |
Source
|
Record name | Resolvin D3 | |
Source | EPA DSSTox | |
URL | https://comptox.epa.gov/dashboard/DTXSID801345702 | |
Description | DSSTox provides a high quality public chemistry resource for supporting improved predictive toxicology. | |
Haftungsausschluss und Informationen zu In-Vitro-Forschungsprodukten
Bitte beachten Sie, dass alle Artikel und Produktinformationen, die auf BenchChem präsentiert werden, ausschließlich zu Informationszwecken bestimmt sind. Die auf BenchChem zum Kauf angebotenen Produkte sind speziell für In-vitro-Studien konzipiert, die außerhalb lebender Organismen durchgeführt werden. In-vitro-Studien, abgeleitet von dem lateinischen Begriff "in Glas", beinhalten Experimente, die in kontrollierten Laborumgebungen unter Verwendung von Zellen oder Geweben durchgeführt werden. Es ist wichtig zu beachten, dass diese Produkte nicht als Arzneimittel oder Medikamente eingestuft sind und keine Zulassung der FDA für die Vorbeugung, Behandlung oder Heilung von medizinischen Zuständen, Beschwerden oder Krankheiten erhalten haben. Wir müssen betonen, dass jede Form der körperlichen Einführung dieser Produkte in Menschen oder Tiere gesetzlich strikt untersagt ist. Es ist unerlässlich, sich an diese Richtlinien zu halten, um die Einhaltung rechtlicher und ethischer Standards in Forschung und Experiment zu gewährleisten.