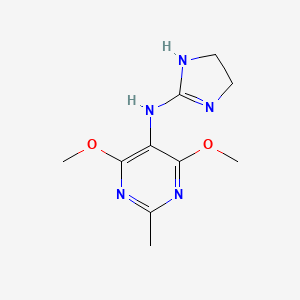
4-Methoxy Moxonidine
Übersicht
Beschreibung
4-Methoxy Moxonidine (CAS 75439-01-9) is a pyrimidine derivative with the molecular formula C₁₀H₁₅N₅O₂ and a molecular weight of 237.26 g/mol . It is recognized as a reference standard and impurity (Moxonidine Impurity B) in pharmaceutical quality control, particularly for the antihypertensive drug Moxonidine . Structurally, it features a pyrimidine core substituted with methoxy, methyl, and imidazoline groups, which influence its physicochemical and biological properties. While its direct therapeutic role is unclear, its structural similarity to Moxonidine makes it critical for analytical and pharmacokinetic studies.
Vorbereitungsmethoden
Classical Synthesis Routes
Early Alkali Metal Hydroxide-Based Methods
The foundational synthesis of 4-methoxy moxonidine, as disclosed in US Patent 4,323,570, involves the reaction of 4,6-dichloro-2-methyl-5-(1-acetyl-2-imidazolin-2-yl)aminopyrimidine (DMAIA) with sodium methoxide in methanol under reflux conditions . This method, while effective, faced criticism due to the corrosive and toxic nature of sodium methoxide, which complicates large-scale production. The reaction proceeds via nucleophilic substitution at the 6-position chlorine atom, replacing it with a methoxy group. The product was isolated via crystallization from nitromethane, yielding 70–75% purity with residual solvent challenges .
Limitations of Early Methods
The use of sodium methoxide necessitated stringent safety protocols and generated byproducts such as acetylated imidazoline derivatives. Furthermore, the crystallization step from nitromethane introduced scalability issues due to the solvent’s toxicity and high cost . These limitations spurred the development of alternative synthetic pathways.
Improved Synthetic Strategies
Organic Base-Mediated Synthesis
A significant advancement emerged with the adoption of organic bases such as potassium carbonate, as detailed in WO2014037391A1 . This method involves suspending DMAIA in methanol with 1.85–2.05 equivalents of potassium carbonate at 65°C for 3–6 hours. The mild base facilitates selective substitution at the 6-position chlorine while minimizing side reactions. Post-reaction, acetic acid and water are added to quench excess base, followed by concentration and precipitation with ammonium hydroxide. This approach achieves an 82% yield with >98% purity, as demonstrated in Example 10 of the patent .
Table 1: Comparison of Base Systems in this compound Synthesis
Base | Solvent | Temperature (°C) | Yield (%) | Purity (%) | Source |
---|---|---|---|---|---|
Sodium methoxide | Methanol | 65 (reflux) | 70–75 | 85–90 | |
Potassium carbonate | Methanol | 65 | 82 | 98 | |
Sodium carbonate | Methanol | 60–65 | 78 | 97 |
Stepwise Addition Protocols
To address the exothermic nature of the reaction, WO2014140034A1 introduced a stepwise addition of DMAIA to a suspension of weak bases in methanol . By dividing DMAIA into 8–10 portions and maintaining temperatures between 60°C and 65°C, this method prevents localized overheating and reduces the formation of impurities such as the "overreacted" byproduct (Impurity C). Cooling the mixture to <25°C within 20 minutes post-reaction further enhances product stability, yielding 85% with <1% impurities .
Solvent and Purification Innovations
Solvent Optimization
The choice of solvent critically influences reaction efficiency. While methanol remains the primary solvent due to its compatibility with DMAIA, mixtures with dimethyl sulfoxide (DMSO) have been explored to enhance solubility. For instance, EP1982983NWB1 reports a 95% yield when using a methanol-DMSO (9:1) system with sodium methoxide at ambient temperature . However, DMSO introduces challenges in subsequent purification steps, necessitating additional washes with ethyl acetate .
Crystallization and Recrystallization
Post-synthesis purification often involves crystallization from nitromethane or ester solvents. A notable improvement, per EP2586779A1, employs ethyl acetate for recrystallization . Crude moxonidine is dissolved in heated ethyl acetate, cooled to 0–5°C, and filtered to remove acetylated impurities. This step elevates purity from 92% to 99.5%, meeting pharmacopeial standards .
Table 2: Purification Methods and Outcomes
Method | Solvent | Temperature (°C) | Purity Improvement (%) | Source |
---|---|---|---|---|
Nitromethane | Nitromethane | 20–25 | 85 → 93 | |
Ethyl acetate | Ethyl acetate | 0–5 | 92 → 99.5 | |
Acetic acid/ammonium | Water | 50 | 80 → 95 |
Mechanistic Insights and Byproduct Formation
Reaction Mechanism
The substitution at the 6-position chlorine proceeds via an SNAr (nucleophilic aromatic substitution) mechanism, where the methoxide ion attacks the electron-deficient pyrimidine ring. The imidazoline moiety acts as an electron-withdrawing group, activating the ring for substitution . Kinetic studies reveal that reaction rates double with every 10°C increase in temperature, but exceeding 70°C accelerates hydrolysis of the acetyl group on the imidazoline, generating Impurity D .
Byproduct Mitigation
Key impurities include:
-
Impurity C : Formed via over-reaction at the 4-position chlorine.
-
Impurity D : Resulting from acetyl group hydrolysis.
Stepwise DMAIA addition and strict temperature control (<65°C) reduce Impurity C to <0.5%, while using anhydrous methanol minimizes Impurity D .
Industrial-Scale Considerations
Environmental and Economic Factors
Modern processes prioritize green chemistry principles. Methanol recovery systems, as described in WO2014037391A1, enable >90% solvent reuse, reducing waste . Additionally, substituting sodium methoxide with potassium carbonate lowers corrosive waste generation, aligning with EPA guidelines .
Regulatory Compliance
The European Pharmacopoeia mandates <0.1% for individual impurities in moxonidine APIs. The ethyl acetate recrystallization method (EP2586779A1) consistently meets this threshold, ensuring compliance .
Analyse Chemischer Reaktionen
Types of Reactions: 4-Methoxy Moxonidine undergoes various chemical reactions, including:
Oxidation: The compound can be oxidized using agents like hydrogen peroxide, leading to the formation of oxidized derivatives.
Reduction: Reduction reactions can be carried out using reducing agents such as sodium borohydride.
Substitution: The methoxy group can be substituted with other functional groups under specific conditions.
Common Reagents and Conditions:
Oxidation: Hydrogen peroxide, catalysts like phosphotungstic acid.
Reduction: Sodium borohydride, lithium aluminum hydride.
Substitution: Halogenating agents, nucleophiles.
Major Products: The major products formed from these reactions depend on the specific reagents and conditions used. For example, oxidation may yield hydroxylated derivatives, while substitution reactions can produce a variety of functionalized compounds .
Wissenschaftliche Forschungsanwendungen
Treatment of Atherosclerosis
Research indicates that moxonidine can play a significant role in treating atherosclerosis. A patent (US5977121A) outlines its use in reducing cholesterol accumulation induced by atherogenic serum and inhibiting the proliferation of cells from atherosclerotic plaques . The findings suggest that moxonidine may help mitigate the progression of atherosclerosis, which is critical given its status as a leading cause of ischemic heart disease.
Management of Metabolic Syndrome
The MERSY study investigated the effects of moxonidine on patients with uncontrolled essential hypertension and metabolic syndrome. This multinational study revealed that treatment with moxonidine led to significant reductions in both systolic and diastolic blood pressure (average reductions of 24.5 mmHg and 12.6 mmHg, respectively) over a six-month period . Furthermore, improvements were noted in metabolic parameters such as body weight and fasting plasma glucose levels, indicating its potential as a multi-faceted treatment option for patients with metabolic syndrome .
Table 1: Effects of Moxonidine on Blood Pressure and Metabolic Parameters
Parameter | Baseline Value | Value After Treatment | Change |
---|---|---|---|
Systolic Blood Pressure | Average ± SD | Average ± SD | -24.5 ± 14.3 mmHg |
Diastolic Blood Pressure | Average ± SD | Average ± SD | -12.6 ± 9.1 mmHg |
Body Weight | Average ± SD | Average ± SD | -2.1 ± 5.4 kg |
Fasting Plasma Glucose | 6.8 mmol/L | 6.2 mmol/L | -0.6 mmol/L |
Triglycerides | 2.4 mmol/L | 2.0 mmol/L | -0.4 mmol/L |
Note: Values are based on findings from the MERSY study involving a diverse population over six months .
Case Studies
Several case studies have documented the clinical efficacy of moxonidine in managing hypertension and metabolic syndrome:
- Case Study 1: A cohort of hypertensive patients treated with moxonidine exhibited not only reduced blood pressure but also favorable changes in lipid profiles and weight management, suggesting an overall reduction in cardiovascular risk factors.
- Case Study 2: In another study involving older adults with metabolic syndrome, moxonidine was effective as an adjunct therapy when traditional antihypertensive medications failed, highlighting its utility in complex patient populations.
Wirkmechanismus
4-Methoxy Moxonidine exerts its effects primarily through its action on imidazoline receptors. It is a selective agonist at the imidazoline receptor subtype 1 (I1), which is found in the rostral ventro-lateral pressor and ventromedial depressor areas of the medulla oblongata. By binding to these receptors, this compound decreases sympathetic nervous system activity, leading to a reduction in blood pressure. Additionally, it may promote sodium excretion, improve insulin resistance, and protect against hypertensive target organ damage .
Vergleich Mit ähnlichen Verbindungen
Comparative Analysis with Structurally and Functionally Related Compounds
Structural Analogues
Moxonidine (Physiotens)
- Molecular Formula : C₉H₁₂ClN₅O (hydrochloride form: C₉H₁₃Cl₂N₅O) .
- Key Features : Central imidazoline I1 receptor agonist with partial α2-adrenergic receptor activity. The 4-methoxy and 2-methyl groups on the pyrimidine ring enhance receptor selectivity .
- Pharmacokinetics : Half-life of ~2.2 hours but effective as a once-daily antihypertensive due to sustained receptor binding .
- Clinical Efficacy: Comparable blood pressure reduction to enalapril, atenolol, and hydrochlorothiazide but with fewer side effects (e.g., dry mouth, sedation) than clonidine .
Clonidine
- Key Features : Dichlorophenyl-imidazoline structure with strong α2-adrenergic receptor affinity.
- Pharmacodynamics : Higher incidence of rebound hypertension and sedation compared to Moxonidine .
- Comparative Data : In gastric acid suppression studies, Moxonidine (ED₅₀: 0.04 mg/kg) demonstrated superior potency and mucosal protection versus clonidine .
Moxonidine-d4
- Molecular Formula : C₉H₈D₄ClN₅O .
- Role : Deuterated analog used as an internal standard in mass spectrometry to quantify Moxonidine and its metabolites in biological samples.
Pyridine/Pyrimidine Derivatives
- Example : 2-Chloro-4-methoxy-6-[(4-methoxyphenyl)methoxy]pyridine () shares a pyridine core with 4-Methoxy Moxonidine but differs in substituents, leading to distinct bioactivity.
- SAR Insight : Methoxy groups at position 4 in indole derivatives (e.g., 4-methoxyindole) enhance antioxidant activity, suggesting similar substituents in Moxonidine derivatives may optimize therapeutic effects .
Functional Analogues (Antihypertensives)
Research Findings and Key Comparisons
Efficacy in Hypertension Management
- Moxonidine vs Clonidine : In a crossover trial, Moxonidine achieved comparable blood pressure control with fewer side effects (30% vs. 85% incidence) .
- Moxonidine vs Enalapril : Placebo-controlled trials showed similar efficacy, but Moxonidine’s central mechanism avoids ACE inhibitor-associated cough .
Gastroprotective Effects
- Moxonidine (1.0 mg/kg) suppressed gastric acid secretion by 100% in rats, outperforming clonidine (46% inhibition at 1.0 mg/kg) .
- The 4-methoxy group may enhance mucosal protection by modulating oxidative stress pathways, as seen in methoxy-substituted antioxidants .
Structural-Activity Relationship (SAR) Insights
- 4-Methoxy Group : Enhances receptor binding and metabolic stability. In pyrazolo[1,5-a]pyridine derivatives, 4-methoxy substitution improves pharmacokinetic profiles .
- Imidazoline Moiety : Critical for I1 receptor selectivity. Removal or modification reduces antihypertensive potency .
- Methyl Groups : Position 2-methyl on the pyrimidine ring may reduce off-target interactions, minimizing side effects .
Biologische Aktivität
4-Methoxy Moxonidine, a derivative of the antihypertensive agent moxonidine, exhibits significant biological activity primarily through its interaction with imidazoline receptors. This article reviews the compound's biological effects, mechanisms of action, pharmacokinetics, and potential therapeutic applications based on diverse research findings.
This compound acts primarily as a selective agonist at the imidazoline receptor subtype 1 (I1). This receptor is predominantly located in the central nervous system, particularly in the rostral ventrolateral medulla, where it modulates sympathetic nervous system (SNS) activity. The activation of I1 receptors leads to:
- Decreased sympathetic outflow , resulting in lower blood pressure.
- Inhibition of peripheral alpha-adrenergic tone , which contributes to vasodilation and reduced systemic vascular resistance .
Biochemical Pathways
The interaction with the I1 receptor influences various biochemical pathways:
- Sympathetic Nervous System Modulation : Reduces catecholamine release, leading to decreased heart rate and blood pressure.
- Metabolic Effects : Enhances insulin sensitivity and reduces leptin levels in obese patients, potentially aiding in weight management .
Pharmacokinetics
This compound demonstrates favorable pharmacokinetic properties:
- Bioavailability : Approximately 88%.
- Metabolism : Primarily occurs in the liver (10-20%).
- Excretion : Predominantly renal (90%) with minimal involvement of cytochrome P450 enzymes .
Antihypertensive Activity
Clinical studies have demonstrated that moxonidine effectively lowers blood pressure in hypertensive patients. A notable study involving 4118 patients reported an average reduction of 24.5 mmHg in systolic blood pressure and 12.6 mmHg in diastolic blood pressure after treatment with moxonidine for six months .
Neuroprotective and Analgesic Effects
Research indicates potential neuroprotective properties of this compound. It may help mitigate neuronal damage in conditions such as ischemia and neurodegenerative diseases due to its ability to regulate SNS activity . Additionally, analgesic effects have been suggested, warranting further investigation into its use for pain management.
Metabolic Syndrome
Moxonidine has shown promise in addressing components of metabolic syndrome, including obesity and insulin resistance. A multicenter study indicated improvements in glycemic control and reductions in body weight among patients treated with moxonidine .
Case Studies
Several case studies highlight the efficacy of moxonidine in diverse populations:
- A study involving patients with metabolic syndrome showed significant reductions in blood pressure and improvements in metabolic parameters after six months of treatment .
- Another study focused on patients with chronic kidney disease revealed that adjunctive moxonidine therapy reduced urine albumin excretion and improved renal function markers .
Comparative Analysis
To better understand the unique properties of this compound compared to other antihypertensive agents, a comparative analysis is presented below:
Compound Name | Mechanism of Action | Unique Features |
---|---|---|
Moxonidine | I1 receptor agonist | Effective for hypertension and metabolic syndrome |
Clonidine | Agonist at I1 and α2 receptors | Used for hypertension; also treats ADHD |
Guanfacine | Selective α2 adrenergic agonist | Primarily for ADHD treatment |
Apraclonidine | Selective α2 receptor agonist | Used for glaucoma management |
Q & A
Basic Research Questions
Q. What is the primary mechanism of action of 4-Methoxy Moxonidine in lowering blood pressure, and how can this be experimentally validated?
this compound acts as a selective agonist for imidazoline I1 receptors in the rostral ventrolateral medulla (RVLM), reducing sympathetic nervous system activity and peripheral vascular resistance . To validate this, researchers can:
- Use in vitro receptor binding assays with radiolabeled ligands (e.g., [³H]-moxonidine) to quantify affinity for I1 receptors versus α2-adrenoceptors .
- Employ genetic models such as α2A-adrenergic receptor (α2A-AR) knockout mice to isolate I1-specific effects .
- Measure changes in plasma norepinephrine levels and renal sympathetic nerve activity in conscious hypertensive rats (e.g., SHRs) after systemic administration .
Q. Which experimental models are most suitable for studying the antihypertensive effects of this compound?
- Spontaneously Hypertensive Rats (SHRs): Chronic dosing studies (e.g., 0.1–1.0 mg/kg/day for 4–8 weeks) can assess long-term blood pressure reduction and regression of end-organ damage (e.g., left ventricular hypertrophy) .
- Pylorus-Ligated Rats: To evaluate peripheral effects on gastric acid secretion, measure basal acid output (BAO) and pepsin levels after intraperitoneal administration (ED50 = 0.04 mg/kg) .
- Conscious Normotensive Models (e.g., Wistar-Kyoto rats): Compare renal responses (diuresis, natriuresis) to acute intravenous doses (0.1–1.0 mg/kg) using metabolic cages and urinary cGMP assays .
Q. What safety protocols are critical when handling this compound in laboratory settings?
- Use negative pressure ventilation for dust/fume control and wear PPE (nitrile gloves, lab coats, face shields) to prevent dermal/ocular exposure .
- Avoid ethanol-induced experimental models without proper waste containment due to compound interactions .
- Monitor cardiovascular parameters (e.g., heart rate, blood pressure) in in vivo studies to detect over-inhibition of sympathetic activity .
Advanced Research Questions
Q. How can researchers resolve contradictions in reported receptor selectivity ratios (I1 vs. α2-adrenoceptors) for this compound?
Discrepancies in selectivity (e.g., 40-fold in some studies vs. 600-fold in others ) may arise from tissue-specific receptor expression or assay conditions. To address this:
- Perform comparative binding assays across tissues (e.g., RVLM, kidney, gastric mucosa) using standardized protocols (e.g., [³⁵S]-GTPγS binding for functional activity) .
- Use imidazoline receptor antagonists (e.g., AGN192403) to isolate I1-mediated effects in dose-response studies .
- Apply computational modeling (e.g., molecular docking simulations) to predict binding affinities under varying pH and ionic conditions .
Q. What methodological approaches distinguish central vs. peripheral contributions to this compound’s anti-secretory effects in the gastrointestinal tract?
- Central Pathway Inhibition: Administer the drug intracerebroventricularly (ICV) in SHRs and measure gastric acid output via continuous pH monitoring .
- Peripheral Action Analysis: Use isolated gastric mucosa preparations ex vivo and quantify bicarbonate secretion via pH-stat titration .
- Genetic Models: Compare effects in α2A-AR−/− mice (minimal central α2 effects) with wild-type controls to isolate I1-mediated peripheral actions .
Q. How does this compound modulate nitric oxide (NO) signaling in the RVLM, and what techniques quantify this interaction?
- iNOS Inhibition Studies: Pretreat rats with S-methylisothiourea (SMT, 3–10 mg/kg IV) and measure attenuation of moxonidine-induced hypotension via arterial catheterization .
- Immunohistochemistry: Quantify iNOS protein upregulation in RVLM tissue sections after chronic ICV infusion (e.g., 14 days) .
- Microdialysis: Collect RVLM extracellular fluid to measure NO metabolites (e.g., nitrite/nitrate) via HPLC .
Q. Data Analysis and Interpretation
Q. How should researchers address variability in dose-response relationships across studies (e.g., ED50 discrepancies in gastric vs. cardiovascular effects)?
- Apply nonlinear mixed-effects modeling (NONMEM) to pool data from multiple studies and identify covariates (e.g., renal function, species differences) .
- Use Bayesian hierarchical models to account for inter-study variability in meta-analyses .
- Validate findings with in vitro-in vivo correlation (IVIVC) using isolated receptor assays and whole-animal pharmacokinetic profiles .
Q. What statistical methods are recommended for analyzing small-sample pharmacodynamic data (e.g., n=8 in gastric studies)?
- Repeated-Measures ANOVA: Assess time-dependent changes in gastric acid output or blood pressure with post-hoc Tukey tests .
- Error Bar Graphs with SEM: Visualize data variability in dose-response curves (e.g., Figure 1 in ).
- Power Analysis: Predefine sample sizes using pilot data (e.g., G*Power software) to ensure ≥80% power for detecting 30% effect sizes .
Q. Contradictory Findings and Mitigation
Q. Why does this compound reduce ethanol-induced gastric injury in rats but exacerbate similar damage in clonidine studies?
- Receptor Selectivity: Moxonidine’s I1 agonism enhances mucosal defense mechanisms (e.g., bicarbonate secretion), whereas clonidine’s α2-adrenoceptor activation may impair mucosal blood flow .
- Experimental Design: Standardize ethanol concentration (e.g., 40% v/v) and pre-treatment intervals (e.g., 30 minutes) across studies .
Q. How can researchers reconcile conflicting reports on moxonidine’s role in heart failure (e.g., mortality risks vs. hemodynamic benefits)?
- Population Pharmacokinetics: Stratify patients by renal function (eGFR <30 mL/min requires dose reduction) to avoid toxicity .
- Biomarker Monitoring: Track plasma ANP and BNP levels to assess cardiac load in preclinical models .
- Avoid Off-Target Effects: Use low doses (≤0.4 mg/day) in hypertension studies to minimize adverse events .
Eigenschaften
IUPAC Name |
N-(4,5-dihydro-1H-imidazol-2-yl)-4,6-dimethoxy-2-methylpyrimidin-5-amine | |
---|---|---|
Source | PubChem | |
URL | https://pubchem.ncbi.nlm.nih.gov | |
Description | Data deposited in or computed by PubChem | |
InChI |
InChI=1S/C10H15N5O2/c1-6-13-8(16-2)7(9(14-6)17-3)15-10-11-4-5-12-10/h4-5H2,1-3H3,(H2,11,12,15) | |
Source | PubChem | |
URL | https://pubchem.ncbi.nlm.nih.gov | |
Description | Data deposited in or computed by PubChem | |
InChI Key |
GFOAVLDLJHAHLM-UHFFFAOYSA-N | |
Source | PubChem | |
URL | https://pubchem.ncbi.nlm.nih.gov | |
Description | Data deposited in or computed by PubChem | |
Canonical SMILES |
CC1=NC(=C(C(=N1)OC)NC2=NCCN2)OC | |
Source | PubChem | |
URL | https://pubchem.ncbi.nlm.nih.gov | |
Description | Data deposited in or computed by PubChem | |
Molecular Formula |
C10H15N5O2 | |
Source | PubChem | |
URL | https://pubchem.ncbi.nlm.nih.gov | |
Description | Data deposited in or computed by PubChem | |
Molecular Weight |
237.26 g/mol | |
Source | PubChem | |
URL | https://pubchem.ncbi.nlm.nih.gov | |
Description | Data deposited in or computed by PubChem | |
Haftungsausschluss und Informationen zu In-Vitro-Forschungsprodukten
Bitte beachten Sie, dass alle Artikel und Produktinformationen, die auf BenchChem präsentiert werden, ausschließlich zu Informationszwecken bestimmt sind. Die auf BenchChem zum Kauf angebotenen Produkte sind speziell für In-vitro-Studien konzipiert, die außerhalb lebender Organismen durchgeführt werden. In-vitro-Studien, abgeleitet von dem lateinischen Begriff "in Glas", beinhalten Experimente, die in kontrollierten Laborumgebungen unter Verwendung von Zellen oder Geweben durchgeführt werden. Es ist wichtig zu beachten, dass diese Produkte nicht als Arzneimittel oder Medikamente eingestuft sind und keine Zulassung der FDA für die Vorbeugung, Behandlung oder Heilung von medizinischen Zuständen, Beschwerden oder Krankheiten erhalten haben. Wir müssen betonen, dass jede Form der körperlichen Einführung dieser Produkte in Menschen oder Tiere gesetzlich strikt untersagt ist. Es ist unerlässlich, sich an diese Richtlinien zu halten, um die Einhaltung rechtlicher und ethischer Standards in Forschung und Experiment zu gewährleisten.