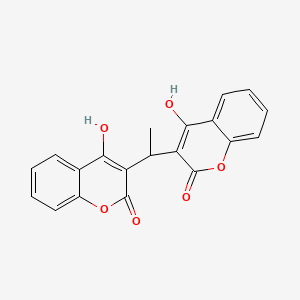
Ethylidene dicoumarol
- Klicken Sie auf QUICK INQUIRY, um ein Angebot von unserem Expertenteam zu erhalten.
- Mit qualitativ hochwertigen Produkten zu einem WETTBEWERBSFÄHIGEN Preis können Sie sich mehr auf Ihre Forschung konzentrieren.
Übersicht
Beschreibung
Äthyli-dicoumarol ist eine coumarinähnliche Verbindung, die für ihre gerinnungshemmende Wirkung bekannt ist. Äthyli-dicoumarol wurde auf seine potenziellen therapeutischen Anwendungen untersucht, insbesondere im Bereich der Gerinnungshemmung.
Vorbereitungsmethoden
Äthyli-dicoumarol kann durch verschiedene Verfahren synthetisiert werden. Ein gängiger Syntheseweg beinhaltet die Reaktion von 4-Hydroxycoumarin mit Aldehydderivaten in Gegenwart eines Katalysators. Zum Beispiel wurde Zn(Prolin)2 als wiederverwendbarer Lewis-Säure-Katalysator für die Herstellung von Dicoumarol-Derivaten verwendet . Die Reaktionsbedingungen umfassen typischerweise milde Temperaturen und ein Lösungsmittel wie Ethanol oder Methanol. Industrielle Produktionsverfahren können eine großtechnische Synthese unter ähnlichen Reaktionsbedingungen umfassen, die jedoch für höhere Ausbeuten und Reinheit optimiert sind.
Analyse Chemischer Reaktionen
Äthyli-dicoumarol unterliegt verschiedenen Arten von chemischen Reaktionen, darunter:
Oxidation: Es kann zu verschiedenen Oxidationsprodukten oxidiert werden.
Reduktion: Reduktionsreaktionen können es in verschiedene reduzierte Formen umwandeln.
Substitution: Es kann Substitutionsreaktionen eingehen, bei denen funktionelle Gruppen durch andere Gruppen ersetzt werden. Häufige Reagenzien, die in diesen Reaktionen verwendet werden, sind Oxidationsmittel wie Wasserstoffperoxid, Reduktionsmittel wie Natriumborhydrid und verschiedene Katalysatoren. Die Hauptprodukte, die aus diesen Reaktionen gebildet werden, hängen von den spezifischen Reagenzien und Bedingungen ab, die verwendet werden.
Wissenschaftliche Forschungsanwendungen
Äthyli-dicoumarol hat verschiedene Anwendungen in der wissenschaftlichen Forschung:
Chemie: Es wird als Modellverbindung zur Untersuchung der Chemie von Coumarinen und deren Derivaten verwendet.
Industrie: Es wird bei der Synthese anderer Coumarin-Derivate und als Reagenz in verschiedenen chemischen Prozessen verwendet.
Wirkmechanismus
Äthyli-dicoumarol entfaltet seine Wirkung durch Hemmung der Vitamin-K-Epoxid-Reduktase, einem Enzym, das am Recycling von Vitamin K beteiligt ist. Diese Hemmung führt zu einem Mangel an aktivem Vitamin K im Blut, was die Bildung von aktivem Prothrombin und anderen Gerinnungsfaktoren verhindert . Zu den molekularen Zielstrukturen gehören Vitamin-K-abhängige Gerinnungsfaktoren wie Prothrombin und Faktoren VII, IX und X.
Wirkmechanismus
Ethylidene dicoumarol exerts its effects by inhibiting vitamin K epoxide reductase, an enzyme involved in the recycling of vitamin K. This inhibition leads to a depletion of active vitamin K in the blood, preventing the formation of active prothrombin and other coagulation factors . The molecular targets include vitamin K-dependent coagulation factors such as prothrombin and factors VII, IX, and X.
Vergleich Mit ähnlichen Verbindungen
Äthyli-dicoumarol ähnelt anderen Coumarin-Derivaten wie Dicoumarol und Warfarin. Es hat jedoch einzigartige Eigenschaften, die es von diesen Verbindungen unterscheiden:
Dicoumarol: Wie Äthyli-dicoumarol ist Dicoumarol ein Gerinnungshemmer, der die Vitamin-K-Epoxid-Reduktase hemmt. Äthyli-dicoumarol kann jedoch unterschiedliche pharmakokinetische Eigenschaften und biologische Aktivitäten aufweisen.
Warfarin: Warfarin ist ein weit verbreiteter Gerinnungshemmer, der ebenfalls die Vitamin-K-Epoxid-Reduktase hemmt. Äthyli-dicoumarol bietet möglicherweise Vorteile hinsichtlich seiner Synthese und des Potenzials, neue Derivate mit verbesserten Eigenschaften zu entwickeln.
Zu den ähnlichen Verbindungen gehören:
- Dicoumarol
- Warfarin
- Andere 4-Hydroxycoumarin-Derivate
Die einzigartige Kombination aus chemischen Eigenschaften und biologischen Aktivitäten von Äthyli-dicoumarol macht es zu einer wertvollen Verbindung für die weitere Forschung und Entwicklung.
Eigenschaften
CAS-Nummer |
1821-16-5 |
---|---|
Molekularformel |
C20H14O6 |
Molekulargewicht |
350.3 g/mol |
IUPAC-Name |
4-hydroxy-3-[1-(4-hydroxy-2-oxochromen-3-yl)ethyl]chromen-2-one |
InChI |
InChI=1S/C20H14O6/c1-10(15-17(21)11-6-2-4-8-13(11)25-19(15)23)16-18(22)12-7-3-5-9-14(12)26-20(16)24/h2-10,21-22H,1H3 |
InChI-Schlüssel |
CPDOMNNHJSTWKL-UHFFFAOYSA-N |
SMILES |
CC(C1=C(C2=CC=CC=C2OC1=O)O)C3=C(C4=CC=CC=C4OC3=O)O |
Kanonische SMILES |
CC(C1=C(C2=CC=CC=C2OC1=O)O)C3=C(C4=CC=CC=C4OC3=O)O |
Herkunft des Produkts |
United States |
Haftungsausschluss und Informationen zu In-Vitro-Forschungsprodukten
Bitte beachten Sie, dass alle Artikel und Produktinformationen, die auf BenchChem präsentiert werden, ausschließlich zu Informationszwecken bestimmt sind. Die auf BenchChem zum Kauf angebotenen Produkte sind speziell für In-vitro-Studien konzipiert, die außerhalb lebender Organismen durchgeführt werden. In-vitro-Studien, abgeleitet von dem lateinischen Begriff "in Glas", beinhalten Experimente, die in kontrollierten Laborumgebungen unter Verwendung von Zellen oder Geweben durchgeführt werden. Es ist wichtig zu beachten, dass diese Produkte nicht als Arzneimittel oder Medikamente eingestuft sind und keine Zulassung der FDA für die Vorbeugung, Behandlung oder Heilung von medizinischen Zuständen, Beschwerden oder Krankheiten erhalten haben. Wir müssen betonen, dass jede Form der körperlichen Einführung dieser Produkte in Menschen oder Tiere gesetzlich strikt untersagt ist. Es ist unerlässlich, sich an diese Richtlinien zu halten, um die Einhaltung rechtlicher und ethischer Standards in Forschung und Experiment zu gewährleisten.