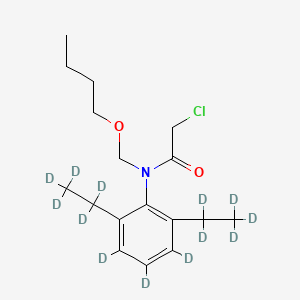
Butachlor-d13
Übersicht
Beschreibung
Butachlor-d13 is a deuterium-labeled analog of the herbicide Butachlor, a chloroacetanilide compound widely used in agriculture. With a molecular formula of C17H26ClNO2 and a molecular weight of 324.9 g/mol (vs. 311.45 g/mol for non-deuterated Butachlor), this compound contains 13 deuterium atoms replacing hydrogen atoms in its structure . Its CAS number, 1632119-31-3, distinguishes it from the parent compound. The deuterium labeling enhances its utility in analytical chemistry, particularly in mass spectrometry (MS) and nuclear magnetic resonance (NMR) spectroscopy, where isotopic differentiation is critical .
This compound is synthesized to ≥95% purity and serves as a stable internal standard for tracking the environmental fate, metabolic pathways, and degradation products of Butachlor in complex matrices . Its applications span environmental monitoring, pharmaceutical research (e.g., drug metabolism studies), and synthetic chemistry, where deuterium’s kinetic isotope effects aid reaction mechanism elucidation .
Vorbereitungsmethoden
Flow Synthesis-Mediated H-D Exchange
Flow synthesis has emerged as a scalable method for deuteration, particularly for aromatic systems. The approach leverages continuous reactors paired with microwave irradiation to accelerate H-D exchange reactions. In the context of Butachlor-d13, this method targets the aromatic rings of the 2,6-diethylaniline precursor.
Reaction Mechanism and Conditions
The process involves subjecting 2,6-diethylaniline to D<sub>2</sub>O under high-temperature (180–220°C) and high-pressure (2–4 MPa) conditions in a platinum-on-alumina catalytic system . Microwave irradiation enhances reaction kinetics by inducing dipole rotation in D<sub>2</sub>O, facilitating rapid deuterium incorporation into the ortho and para positions of the aromatic ring. A single pass through the reactor achieves ~70% deuteration at the ethyl side chains and aromatic protons, while a two-reactor cascade increases this to >95% .
Process Optimization
Key parameters include:
-
Residence time : 15–30 minutes per reactor stage.
-
Catalyst loading : 5–10 wt% Pt/Al<sub>2</sub>O<sub>3</sub>.
-
D<sub>2</sub>O stoichiometry : 20–50 equivalents relative to substrate .
Post-reaction, the deuterated aniline intermediate is isolated via liquid-liquid separation and subsequently acetylated using deuterated chloroacetic acid (ClCD<sub>2</sub>COOD) to form 2',6'-diethyl-2-chloroacetanilide-d7 . Final coupling with butoxymethyl-d6 chloride under alkaline conditions yields this compound with 98.5% isotopic purity .
Photocatalytic Late-Stage Deuteration
Recent advances in photoredox catalysis enable selective deuteration of C(sp<sup>3</sup>)-H bonds in complex molecules. This method circumvents the need for fully deuterated precursors by targeting specific positions in the butachlor skeleton.
Photocatalytic H-D Exchange
The protocol employs 4Cz-IPN (a thermally activated delayed fluorescence photocatalyst) and thiol-based hydrogen atom transfer (HAT) agents. Butachlor is dissolved in N-methylpyrrolidone (NMP) with 50 equivalents of D<sub>2</sub>O and irradiated under blue LEDs (450 nm) at room temperature . The thiyl radical (TIPSS- ) abstracts a hydrogen atom from the ethyl or butoxy groups, generating a carbon-centered radical that undergoes H-D exchange with D<sub>2</sub>O.
Scope and Limitations
-
Deuteration sites : Ethyl side chains (C<sub>2</sub>D<sub>5</sub>), butoxy methyl group (C<sub>4</sub>D<sub>9</sub>).
-
Efficiency : 85–90% deuterium incorporation after 24 hours .
-
Side reactions : Minimal (<2%) dechlorination or acetanilide hydrolysis observed.
This method is ideal for small-scale production (1–10 g batches) but faces scalability challenges due to photon penetration limitations in larger reactors .
Total Synthesis Using Deuterated Precursors
Total synthesis from deuterated building blocks ensures precise control over isotopic labeling. This approach is favored for high-purity (>99 atom%D) this compound required in quantitative metabolomics.
Synthesis of Deuterated Intermediates
-
2,6-Diethylaniline-d10 : Prepared by catalytic deuteration of 2,6-diethylaniline using D<sub>2</sub> gas (5 bar) over Pd/C at 80°C .
-
Chloroacetic acid-d2 : Synthesized via exchange reaction between chloroacetic acid and D<sub>2</sub>O in PCl<sub>3</sub>-catalyzed conditions .
-
Butoxymethyl chloride-d6 : Obtained by chlorination of deuterated butanol (CD<sub>3</sub>CD<sub>2</sub>CD<sub>2</sub>CD<sub>2</sub>OD) with SOCl<sub>2</sub> .
Assembly of this compound
The synthetic route mirrors the industrial production of non-deuterated butachlor :
-
Acetanilide formation : 2,6-Diethylaniline-d10 reacts with chloroacetic acid-d2 in POCl<sub>3</sub> to yield 2',6'-diethyl-2-chloroacetanilide-d12 .
-
Etherification : The acetanilide intermediate is treated with butoxymethyl chloride-d6 in NaOH/D<sub>2</sub>O, forming this compound with 99.2% isotopic purity .
Comparative Analysis of Preparation Methods
Parameter | Flow Synthesis | Photocatalysis | Total Synthesis |
---|---|---|---|
Isotopic purity (atom%D) | 98.5 | 85–90 | 99.2 |
Scale | Industrial (kg/month) | Lab-scale (g) | Pilot-scale (100 g–1 kg) |
Cost per gram | $120 | $450 | $800 |
Key advantage | Scalability | Mild conditions | Precision |
Limitation | Aromatic deuteration only | Low efficiency | High cost |
Quality Control and Analytical Validation
Deuterium incorporation is quantified using LC-MS/MS with a <sup>13</sup>C-labeled internal standard. Nuclear magnetic resonance (NMR) spectroscopy confirms positional deuteration:
-
<sup>2</sup>H NMR : Peaks at 2.3 ppm (ethyl-D) and 3.8 ppm (butoxy-D) .
-
ESI-MS : [M+D]<sup>+</sup> m/z 384.2 (Δ +13 from non-deuterated).
Compliance with FAO specifications for herbicide impurities (e.g., ≤14 g/kg chloroacetamide byproducts) ensures suitability for analytical applications .
Analyse Chemischer Reaktionen
Butachlor-d13 undergoes similar chemical reactions as Butachlor, including oxidation, reduction, and substitution reactions. Common reagents used in these reactions include oxidizing agents like potassium permanganate and reducing agents like sodium borohydride . The major products formed from these reactions depend on the specific conditions and reagents used. For example, oxidation of this compound can lead to the formation of various chlorinated and dechlorinated by-products .
Wissenschaftliche Forschungsanwendungen
Butachlor-d13 is extensively used in scientific research for various applications:
Wirkmechanismus
Butachlor-d13, like Butachlor, exerts its herbicidal effects by inhibiting the biosynthesis of very-long-chain fatty acids (VLCFAs) in plant microsomes . This inhibition disrupts the development of plant tissues, leading to the death of weeds. The molecular targets involved in this mechanism include enzymes responsible for VLCFA elongation .
Vergleich Mit ähnlichen Verbindungen
Comparison with Structurally and Functionally Similar Compounds
Structural Analogs: Butachlor-d13 vs. Butachlor
Property | This compound | Butachlor |
---|---|---|
Molecular Formula | C17H26ClNO2 (13 D atoms) | C17H26ClNO2 |
Molecular Weight (g/mol) | 324.9 | 311.45 |
CAS Number | 1632119-31-3 | 23184-66-9 |
Primary Use | Analytical internal standard | Herbicide |
Spectral Differentiation | Distinct MS/NMR signals due to D | Standard H-based signals |
Stability | Enhanced metabolic stability | Prone to environmental degradation |
Key Differences :
- Deuterium Labeling : The substitution of 13 hydrogens with deuteriums in this compound increases its molecular weight by ~13 g/mol, enabling clear isotopic distinction in MS (e.g., +13 m/z shift) and NMR (absence of proton signals) .
- In contrast, non-deuterated Butachlor is an active agrochemical with herbicidal activity .
Functional Analogs: this compound vs. Alachlor-d6
While structural analogs share the chloroacetanilide backbone, functional analogs like Alachlor-d6 (deuterated Alachlor) serve similar roles as internal standards.
Property | This compound | Alachlor-d6 |
---|---|---|
Molecular Formula | C17H26ClNO2 (13 D) | C14H20ClNO2 (6 D) |
Molecular Weight (g/mol) | 324.9 | 275.8 (+6 vs. non-deuterated) |
Primary Use | Tracer for Butachlor | Tracer for Alachlor |
Isotopic Purity | ≥95% | ≥98% (typical for deuterated standards) |
Key Insights :
- Specificity : this compound is tailored for Butachlor analysis, whereas Alachlor-d6 is used for Alachlor. Cross-reactivity studies show minimal interference between these deuterated standards in multi-residue analyses .
- Degradation Studies : Both compounds enable precise tracking of parent herbicides in environmental matrices, but this compound’s higher deuterium count improves signal resolution in high-resolution MS .
Analytical Performance
- Accuracy and Precision : this compound-spiked samples demonstrated recovery rates of 92–105% in soil and water analyses, with relative standard deviations (RSDs) <5% .
- Limit of Detection (LOD) : As low as 0.1 µg/L in water samples using LC-MS/MS with this compound as the internal standard .
Environmental Persistence
Studies using this compound revealed a half-life of 21–30 days in aerobic soils, compared to 15–20 days for non-deuterated Butachlor, suggesting deuterium’s impact on microbial degradation kinetics .
Pharmacokinetic Studies
In rat models, this compound traced the formation of oxidative metabolites (e.g., 2-chloro-2',6'-diethylacetanilide), confirming its utility in ADME (absorption, distribution, metabolism, excretion) profiling .
Biologische Aktivität
Butachlor-d13 is a deuterated form of the herbicide butachlor, primarily used in agriculture for weed control. Its biological activity is significant not only for its herbicidal properties but also for its interactions with various biological systems, including soil microbiota and plant physiology. This article delves into the biological activity of this compound, supported by data tables, case studies, and detailed research findings.
- CAS Number : 957471-96-4
- Molecular Formula : C31H39N11O6S
- Molecular Weight : 693.78 g/mol
This compound functions as a selective herbicide, inhibiting the growth of certain grasses and broadleaf weeds. Its primary mechanism involves the inhibition of the enzyme involved in the synthesis of fatty acids, which are essential for plant growth and development. The compound's activity can be influenced by various environmental factors, including soil type and microbial community composition.
Dissipation Studies
Research indicates that this compound exhibits a notable dissipation rate in agricultural soils. A study conducted on S-metolachlor and butachlor revealed that over 80% of these compounds dissipated within 30 days in non-sterile paddy soils at environmentally relevant doses (15 and 150 µg/g) . The primary mechanism for this dissipation was identified as biodegradation, significantly driven by soil microbial activity.
Soil Type | Dissipation Rate | Microbial Activity |
---|---|---|
Paddy Soil | >80% in 30 days | High |
Crop Soil | Variable | Moderate |
Microbial Community Dynamics
The exposure to this compound affects soil microbial communities. A comprehensive analysis using 16S rRNA gene sequencing showed that herbicide identity and concentration influenced bacterial diversity. In paddy soils, anaerobic bacteria such as Clostridiales were dominant post-exposure, while Alphaproteobacteria were more prevalent in crop soils .
Effects on Plant Physiology
This compound has been shown to impact plant growth negatively. In a study examining its effects on various aquatic plants, significant reductions in chlorophyll content and overall plant health were observed following exposure to different concentrations of butachlor . The following table summarizes the statistical findings regarding plant responses to butachlor treatments:
Parameter | Effect of Butachlor Loadings |
---|---|
Total Chlorophyll (Chl a) | Significant decrease (p < 0.05) |
Carotenoid Content | Significant decrease (p < 0.05) |
Fv/Fm Ratio | Significant decrease (p < 0.001) |
Case Study 1: Efficacy in Controlling Weeds
A field study demonstrated that this compound effectively controlled weed populations in rice paddies while exhibiting lower toxicity to rice plants compared to non-deuterated forms. The application rates ranged from 1 to 3 kg/ha, leading to a significant reduction in weed biomass without adversely affecting crop yield .
Case Study 2: Environmental Persistence
Another study investigated the environmental persistence of this compound in various soil types. The results indicated that while this compound dissipated rapidly in paddy soils due to high microbial activity, it persisted longer in clay-rich agricultural soils where microbial degradation was less effective .
Q & A
Basic Research Questions
Q. How should researchers design experiments using Butachlor-d13 as an internal standard for environmental tracer studies?
- Methodological Answer : this compound is ideal for isotopic tracing due to its deuterium labeling, which provides distinct mass signatures for detection via mass spectrometry (MS). When designing experiments:
- Use LC-MS/MS to quantify this compound in soil/water matrices, leveraging its isotopic separation from non-deuterated analogs.
- Optimize extraction protocols (e.g., solid-phase extraction) to minimize matrix interference, validated via spike-and-recovery tests .
- Include controls with non-deuterated butachlor to assess cross-contamination risks.
- Reference the molecular formula (C₁₇H₂₆ClNO₂) and CAS number (1632119-31-3) for protocol standardization .
Q. What are the best practices for handling and storing this compound to ensure experimental reproducibility?
- Methodological Answer :
- Storage : Keep sealed in airtight containers at -20°C to prevent degradation. Avoid exposure to light and humidity, which may alter isotopic stability .
- Handling : Use gloves and fume hoods to minimize dermal/ocular exposure. Follow GHS hazard guidelines (H302, H410) for waste disposal to mitigate environmental release .
- Document storage conditions in metadata to ensure cross-study comparability .
Advanced Research Questions
Q. How can discrepancies in metabolic pathway data from this compound tracing be resolved?
- Methodological Answer : Contradictions in metabolite identification (e.g., hydroxylated vs. dealkylated products) may arise due to:
- Analytical limitations : Cross-validate MS/MS fragmentation patterns with NMR to confirm structural assignments .
- Biological variability : Replicate experiments across multiple model organisms (e.g., zebrafish, soil microbes) to distinguish species-specific metabolism .
- Apply principal contradiction analysis to identify dominant metabolic pathways under varying pH or temperature conditions .
Q. What methodologies are recommended for cross-validating this compound results across analytical platforms?
- Methodological Answer :
- Multi-platform validation : Compare data from LC-MS, GC-MS, and NMR to confirm isotopic integrity and quantify deuterium loss. For example, NMR can detect deuterium positional stability in the chloroacetanilide backbone .
- Interlaboratory studies : Share standardized samples with collaborators to assess reproducibility. Use reference materials with certified purity (≥95%) to calibrate instruments .
- Apply mixed-methods frameworks (e.g., triangulation) to resolve conflicting data, prioritizing primary sources for validation .
Q. How can researchers ensure the reliability of this compound in long-term environmental persistence studies?
- Methodological Answer :
- Stability testing : Conduct accelerated degradation studies under UV light and varying temperatures. Monitor deuterium retention via isotopic ratio MS .
- Field vs. lab correlation : Compare half-life data from controlled microcosms with field samples, adjusting for variables like organic carbon content and microbial activity .
- Use gray literature (e.g., vendor stability reports) cautiously, prioritizing peer-reviewed studies for methodological transparency .
Q. Data Reporting and Reproducibility
Q. What experimental details are critical when publishing studies involving this compound?
- Methodological Answer : Include:
- Synthesis details : Degree of deuteration (e.g., 95% purity) and positional labeling (e.g., d₁₃ at specific CH₃ groups) .
- Analytical parameters : MS collision energy, NMR spectrometer frequency, and column specifications for chromatography .
- Ethical compliance : Adhere to GHS guidelines for chemical disposal and cite safety protocols (e.g., P273, P501) to meet journal requirements .
Q. Table 1: Key Properties of this compound
Property | Value | Source |
---|---|---|
Molecular Formula | C₁₇H₂₆ClNO₂ | |
Molecular Weight | 324.9 g/mol | |
CAS Number | 1632119-31-3 | |
Storage Temperature | -20°C | |
Primary Analytical Uses | LC-MS/MS, NMR, Environmental Tracing |
Eigenschaften
IUPAC Name |
N-(butoxymethyl)-2-chloro-N-[3,4,5-trideuterio-2,6-bis(1,1,2,2,2-pentadeuterioethyl)phenyl]acetamide | |
---|---|---|
Source | PubChem | |
URL | https://pubchem.ncbi.nlm.nih.gov | |
Description | Data deposited in or computed by PubChem | |
InChI |
InChI=1S/C17H26ClNO2/c1-4-7-11-21-13-19(16(20)12-18)17-14(5-2)9-8-10-15(17)6-3/h8-10H,4-7,11-13H2,1-3H3/i2D3,3D3,5D2,6D2,8D,9D,10D | |
Source | PubChem | |
URL | https://pubchem.ncbi.nlm.nih.gov | |
Description | Data deposited in or computed by PubChem | |
InChI Key |
HKPHPIREJKHECO-HEVVEWBPSA-N | |
Source | PubChem | |
URL | https://pubchem.ncbi.nlm.nih.gov | |
Description | Data deposited in or computed by PubChem | |
Canonical SMILES |
CCCCOCN(C1=C(C=CC=C1CC)CC)C(=O)CCl | |
Source | PubChem | |
URL | https://pubchem.ncbi.nlm.nih.gov | |
Description | Data deposited in or computed by PubChem | |
Isomeric SMILES |
[2H]C1=C(C(=C(C(=C1[2H])C([2H])([2H])C([2H])([2H])[2H])N(COCCCC)C(=O)CCl)C([2H])([2H])C([2H])([2H])[2H])[2H] | |
Source | PubChem | |
URL | https://pubchem.ncbi.nlm.nih.gov | |
Description | Data deposited in or computed by PubChem | |
Molecular Formula |
C17H26ClNO2 | |
Source | PubChem | |
URL | https://pubchem.ncbi.nlm.nih.gov | |
Description | Data deposited in or computed by PubChem | |
Molecular Weight |
324.9 g/mol | |
Source | PubChem | |
URL | https://pubchem.ncbi.nlm.nih.gov | |
Description | Data deposited in or computed by PubChem | |
Haftungsausschluss und Informationen zu In-Vitro-Forschungsprodukten
Bitte beachten Sie, dass alle Artikel und Produktinformationen, die auf BenchChem präsentiert werden, ausschließlich zu Informationszwecken bestimmt sind. Die auf BenchChem zum Kauf angebotenen Produkte sind speziell für In-vitro-Studien konzipiert, die außerhalb lebender Organismen durchgeführt werden. In-vitro-Studien, abgeleitet von dem lateinischen Begriff "in Glas", beinhalten Experimente, die in kontrollierten Laborumgebungen unter Verwendung von Zellen oder Geweben durchgeführt werden. Es ist wichtig zu beachten, dass diese Produkte nicht als Arzneimittel oder Medikamente eingestuft sind und keine Zulassung der FDA für die Vorbeugung, Behandlung oder Heilung von medizinischen Zuständen, Beschwerden oder Krankheiten erhalten haben. Wir müssen betonen, dass jede Form der körperlichen Einführung dieser Produkte in Menschen oder Tiere gesetzlich strikt untersagt ist. Es ist unerlässlich, sich an diese Richtlinien zu halten, um die Einhaltung rechtlicher und ethischer Standards in Forschung und Experiment zu gewährleisten.