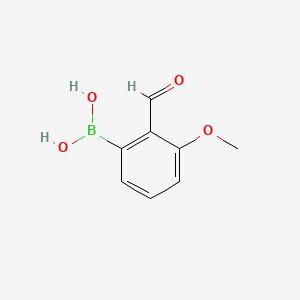
(2-Formyl-3-methoxyphenyl)boronsäure
Übersicht
Beschreibung
(2-Formyl-3-methoxyphenyl)boronic acid is an organic compound with the molecular formula C8H9BO4 It is a boronic acid derivative, characterized by the presence of a formyl group and a methoxy group attached to a phenyl ring
Wissenschaftliche Forschungsanwendungen
(2-Formyl-3-methoxyphenyl)boronic acid has a wide range of applications in scientific research:
Biology: The compound can be used in the development of boron-containing drugs and as a probe for studying biological systems.
Wirkmechanismus
Target of Action
The primary target of (2-Formyl-3-methoxyphenyl)boronic acid is the Suzuki–Miyaura (SM) coupling reaction . This reaction is a widely-applied transition metal catalyzed carbon–carbon bond forming reaction .
Mode of Action
The (2-Formyl-3-methoxyphenyl)boronic acid interacts with its target through two key processes: oxidative addition and transmetalation . In oxidative addition, palladium becomes oxidized through its donation of electrons to form a new Pd–C bond . In transmetalation, the organoboron reagents, which are formally nucleophilic organic groups, are transferred from boron to palladium .
Biochemical Pathways
The compound affects the Suzuki–Miyaura coupling pathway . This pathway involves the conjoining of chemically differentiated fragments that participate in electronically divergent processes with the metal catalyst . The broad application of this pathway arises from the exceptionally mild and functional group tolerant reaction conditions, the relatively stable, readily prepared and generally environmentally benign nature of the organoboron reagents, and their rapid transmetalation with palladium (II) complexes .
Pharmacokinetics
It is known that the compound is used as a reagent in the synthesis of aromatic compounds, drugs, and pesticides .
Result of Action
The molecular and cellular effects of the compound’s action are primarily seen in the formation of carbon–carbon bonds during the Suzuki–Miyaura coupling reaction . This results in the synthesis of a variety of natural products, pharmaceutical targets, and lead compounds .
Action Environment
The action, efficacy, and stability of (2-Formyl-3-methoxyphenyl)boronic acid are influenced by various environmental factors. These include the reaction conditions, the stability of the organoboron reagents, and the speed of transmetalation with palladium (II) complexes . The compound’s success in the Suzuki–Miyaura coupling reaction originates from a combination of exceptionally mild and functional group tolerant reaction conditions .
Biochemische Analyse
Biochemical Properties
Boronic acids are known to have intrinsic affinity for carbohydrates . This suggests that (2-Formyl-3-methoxyphenyl)boronic acid may interact with enzymes, proteins, and other biomolecules that have carbohydrate components.
Molecular Mechanism
Boronic acids are known to participate in Suzuki–Miyaura coupling, a widely-used transition metal catalyzed carbon–carbon bond-forming reaction . This suggests that (2-Formyl-3-methoxyphenyl)boronic acid may exert its effects at the molecular level through similar mechanisms.
Vorbereitungsmethoden
Synthetic Routes and Reaction Conditions: The synthesis of (2-Formyl-3-methoxyphenyl)boronic acid typically involves the borylation of the corresponding aryl halide. One common method is the palladium-catalyzed Suzuki-Miyaura coupling reaction, where an aryl halide reacts with a boronic acid or boronate ester in the presence of a palladium catalyst and a base. The reaction conditions often include the use of solvents such as toluene or ethanol and temperatures ranging from 50°C to 100°C .
Industrial Production Methods: Industrial production of (2-Formyl-3-methoxyphenyl)boronic acid may involve similar synthetic routes but on a larger scale. The process is optimized for higher yields and purity, often employing continuous flow reactors and automated systems to ensure consistent quality and efficiency .
Analyse Chemischer Reaktionen
Types of Reactions: (2-Formyl-3-methoxyphenyl)boronic acid undergoes various chemical reactions, including:
Oxidation: The formyl group can be oxidized to a carboxylic acid using oxidizing agents such as potassium permanganate or chromium trioxide.
Reduction: The formyl group can be reduced to a hydroxymethyl group using reducing agents like sodium borohydride or lithium aluminum hydride.
Common Reagents and Conditions:
Oxidation: Potassium permanganate, chromium trioxide.
Reduction: Sodium borohydride, lithium aluminum hydride.
Substitution: Palladium catalysts, bases like potassium carbonate, solvents like toluene or ethanol.
Major Products:
Oxidation: (2-Formyl-3-methoxyphenyl)carboxylic acid.
Reduction: (2-Hydroxymethyl-3-methoxyphenyl)boronic acid.
Substitution: Various biaryl compounds depending on the coupling partner.
Vergleich Mit ähnlichen Verbindungen
- 3-Methoxyphenylboronic acid
- 4-Methoxyphenylboronic acid
- 2-Formylphenylboronic acid
- 3-Formylphenylboronic acid
- 4-Formylphenylboronic acid
Comparison: (2-Formyl-3-methoxyphenyl)boronic acid is unique due to the presence of both a formyl and a methoxy group on the phenyl ring. This combination of functional groups imparts distinct reactivity and properties compared to its analogs. For instance, the formyl group can participate in additional reactions such as oxidation and reduction, while the methoxy group can influence the electronic properties of the molecule, affecting its reactivity in cross-coupling reactions .
Eigenschaften
IUPAC Name |
(2-formyl-3-methoxyphenyl)boronic acid | |
---|---|---|
Source | PubChem | |
URL | https://pubchem.ncbi.nlm.nih.gov | |
Description | Data deposited in or computed by PubChem | |
InChI |
InChI=1S/C8H9BO4/c1-13-8-4-2-3-7(9(11)12)6(8)5-10/h2-5,11-12H,1H3 | |
Source | PubChem | |
URL | https://pubchem.ncbi.nlm.nih.gov | |
Description | Data deposited in or computed by PubChem | |
InChI Key |
XSAPYCFXQHQPMM-UHFFFAOYSA-N | |
Source | PubChem | |
URL | https://pubchem.ncbi.nlm.nih.gov | |
Description | Data deposited in or computed by PubChem | |
Canonical SMILES |
B(C1=C(C(=CC=C1)OC)C=O)(O)O | |
Source | PubChem | |
URL | https://pubchem.ncbi.nlm.nih.gov | |
Description | Data deposited in or computed by PubChem | |
Molecular Formula |
C8H9BO4 | |
Source | PubChem | |
URL | https://pubchem.ncbi.nlm.nih.gov | |
Description | Data deposited in or computed by PubChem | |
Molecular Weight |
179.97 g/mol | |
Source | PubChem | |
URL | https://pubchem.ncbi.nlm.nih.gov | |
Description | Data deposited in or computed by PubChem | |
Synthesis routes and methods
Procedure details
Haftungsausschluss und Informationen zu In-Vitro-Forschungsprodukten
Bitte beachten Sie, dass alle Artikel und Produktinformationen, die auf BenchChem präsentiert werden, ausschließlich zu Informationszwecken bestimmt sind. Die auf BenchChem zum Kauf angebotenen Produkte sind speziell für In-vitro-Studien konzipiert, die außerhalb lebender Organismen durchgeführt werden. In-vitro-Studien, abgeleitet von dem lateinischen Begriff "in Glas", beinhalten Experimente, die in kontrollierten Laborumgebungen unter Verwendung von Zellen oder Geweben durchgeführt werden. Es ist wichtig zu beachten, dass diese Produkte nicht als Arzneimittel oder Medikamente eingestuft sind und keine Zulassung der FDA für die Vorbeugung, Behandlung oder Heilung von medizinischen Zuständen, Beschwerden oder Krankheiten erhalten haben. Wir müssen betonen, dass jede Form der körperlichen Einführung dieser Produkte in Menschen oder Tiere gesetzlich strikt untersagt ist. Es ist unerlässlich, sich an diese Richtlinien zu halten, um die Einhaltung rechtlicher und ethischer Standards in Forschung und Experiment zu gewährleisten.