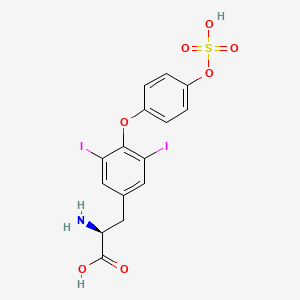
3,5-Diiod-L-thyronin 4'-O-Sulfat
- Klicken Sie auf QUICK INQUIRY, um ein Angebot von unserem Expertenteam zu erhalten.
- Mit qualitativ hochwertigen Produkten zu einem WETTBEWERBSFÄHIGEN Preis können Sie sich mehr auf Ihre Forschung konzentrieren.
Übersicht
Beschreibung
3,5-Diiodo-L-thyronine 4'-O-Sulfate: is a complex organic compound characterized by the presence of amino, diiodo, and sulfooxyphenoxy groups
Wissenschaftliche Forschungsanwendungen
Chemistry
Catalysis: The compound can act as a ligand in catalytic reactions, enhancing reaction rates and selectivity.
Material Science: Used in the synthesis of advanced materials with specific electronic or optical properties.
Biology
Biochemical Probes: Utilized as a probe to study enzyme activities and protein interactions.
Drug Development: Potential precursor for the synthesis of pharmaceutical compounds with therapeutic properties.
Medicine
Diagnostic Agents: Employed in the development of diagnostic agents for imaging techniques.
Therapeutics: Investigated for its potential therapeutic effects in treating certain diseases.
Industry
Dye Synthesis: Used in the production of dyes with specific color properties.
Polymer Additives: Incorporated into polymers to enhance their stability and performance.
Wirkmechanismus
Target of Action
The primary target of 3,5-Diiodo-L-thyronine 4’-O-Sulfate, also known as (2S)-2-Amino-3-[3,5-diiodo-4-(4-sulfooxyphenoxy)phenyl]propanoic acid, is the mitochondria . It is an iodinated thyronine hormone that regulates gene activity affecting processes such as homeostasis, lipid metabolism, and insulin resistance .
Mode of Action
3,5-Diiodo-L-thyronine 4’-O-Sulfate interacts with its targets primarily through Thyroid Hormone Receptors (THRs)-independent ways , with mitochondria as a likely cellular target . Thrs-mediated actions have also been described . It has been reported that 3,5-Diiodo-L-thyronine represses THRβ expression and impairs its up-regulation by cortisol possibly through a transrepression mechanism .
Biochemical Pathways
The compound affects various biochemical pathways, particularly those related to energy metabolism . It has been shown to rapidly increase resting metabolic rate and elicit short-term beneficial hypolipidemic effects .
Pharmacokinetics
It is known that the compound’s effects on homeostasis, lipid metabolism, and insulin resistance suggest that it may have significant bioavailability .
Result of Action
The molecular and cellular effects of 3,5-Diiodo-L-thyronine 4’-O-Sulfate’s action include the regulation of gene activity affecting processes such as homeostasis, lipid metabolism, and insulin resistance . In rodent models, it has been shown to rapidly increase resting metabolic rate and elicit short-term beneficial hypolipidemic effects .
Biochemische Analyse
Biochemical Properties
3,5-Diiodo-L-thyronine 4’-O-Sulfate exhibits interesting metabolic activities . It stimulates the TR-beta receptor for thyroid hormones, thus increasing energy expenditure . It has agonistic effects at myocardial tissue and pituitary, which results in 3,5-Diiodo-L-thyronine 4’-O-Sulfate suppressing TSH release .
Cellular Effects
3,5-Diiodo-L-thyronine 4’-O-Sulfate influences cell function by modulating energy metabolism . It rapidly increases resting metabolic rate and elicits short-term beneficial hypolipidemic effects .
Molecular Mechanism
3,5-Diiodo-L-thyronine 4’-O-Sulfate acts through Thyroid hormone receptors (THRs)-independent ways, with mitochondria as a likely cellular target . Thrs-mediated actions have also been described .
Temporal Effects in Laboratory Settings
In rodent models, exogenously administered 3,5-Diiodo-L-thyronine 4’-O-Sulfate rapidly increases resting metabolic rate . Further analyses on larger cohorts are needed to determine whether 3,5-Diiodo-L-thyronine 4’-O-Sulfate is a potent additional modulator of energy metabolism .
Dosage Effects in Animal Models
The effects of 3,5-Diiodo-L-thyronine 4’-O-Sulfate vary with different dosages in animal models .
Metabolic Pathways
3,5-Diiodo-L-thyronine 4’-O-Sulfate is involved in the thyroid hormone metabolic pathway . It interacts with various enzymes and cofactors, affecting metabolic flux or metabolite levels .
Vorbereitungsmethoden
Synthetic Routes and Reaction Conditions
The synthesis of 3,5-Diiodo-L-thyronine 4'-O-Sulfate typically involves multiple steps:
Sulfonation: The sulfooxy group is introduced by reacting the phenol derivative with sulfur trioxide or chlorosulfonic acid.
Amino Acid Coupling: The final step involves coupling the iodinated and sulfonated phenyl derivative with a suitable amino acid precursor under controlled conditions to ensure the correct stereochemistry.
Industrial Production Methods
Industrial production of this compound may involve:
Batch Processing: Sequential addition of reagents in a controlled environment to ensure high purity and yield.
Continuous Flow Synthesis: Utilizing continuous flow reactors to enhance reaction efficiency and scalability.
Analyse Chemischer Reaktionen
Types of Reactions
Oxidation: The compound can undergo oxidation reactions, particularly at the amino group, forming various oxidized derivatives.
Reduction: Reduction reactions can target the iodine atoms, potentially leading to deiodinated products.
Substitution: The sulfooxy group can be substituted with other functional groups through nucleophilic substitution reactions.
Common Reagents and Conditions
Oxidation: Common oxidizing agents include hydrogen peroxide and potassium permanganate.
Reduction: Reducing agents such as sodium borohydride or lithium aluminum hydride are used.
Substitution: Nucleophiles like amines or thiols can be employed under basic conditions.
Major Products
Oxidized Derivatives: Formation of nitroso or nitro derivatives.
Deiodinated Products: Compounds with fewer iodine atoms.
Substituted Products: Various derivatives depending on the nucleophile used.
Vergleich Mit ähnlichen Verbindungen
Similar Compounds
4,5-Dihydroxy-1,3-benzenedisulfonic acid: Shares the sulfooxyphenoxy group but lacks the amino and diiodo groups.
4,4’-Difluorobenzophenone: Contains a similar phenyl structure but with fluorine atoms instead of iodine.
Uniqueness
Structural Complexity: The presence of both diiodo and sulfooxyphenoxy groups in a single molecule is unique.
Functional Versatility:
Biologische Aktivität
3,5-Diiodo-L-thyronine 4'-O-sulfate (often referred to as 3,5-T2 sulfate) is a sulfated derivative of the thyroid hormone metabolite 3,5-diiodo-L-thyronine (3,5-T2). This compound has garnered attention in recent years due to its potential biological activities and implications for metabolic regulation. This article explores the biological activity of 3,5-T2 sulfate, focusing on its mechanisms of action, effects on cellular metabolism, and potential therapeutic applications.
The biological activity of 3,5-T2 sulfate is primarily mediated through its interaction with thyroid hormone receptors and other cellular targets. It operates via both genomic and non-genomic pathways:
- Genomic Pathways : 3,5-T2 sulfate binds to thyroid hormone receptors in the nucleus, influencing gene expression related to metabolism and energy expenditure.
- Non-Genomic Pathways : The compound affects mitochondrial function and oxidative stress, modulating energy metabolism through rapid signaling mechanisms.
These interactions suggest that 3,5-T2 sulfate may play a role in regulating metabolic processes more rapidly than traditional thyroid hormones like thyroxine (T4) and triiodothyronine (T3) .
Biological Effects
Research indicates that 3,5-T2 sulfate influences various physiological processes:
- Mitochondrial Function : Studies have shown that 3,5-T2 sulfate enhances mitochondrial oxidative capacity. For instance, it has been reported to increase fatty acid uptake and oxidation rates in tissues such as liver, skeletal muscle, and brown adipose tissue .
- Metabolic Regulation : The compound has been linked to improved metabolic outcomes in animal models. For example, it demonstrated potential in reducing hepatic steatosis (fatty liver disease) by modulating energy metabolism pathways differently than T3 .
- Cold Tolerance : In hypothyroid rat models, treatment with 3,5-T2 sulfate improved cold tolerance and survival rates during cold exposure .
Comparative Biological Activity
The following table summarizes the biological activities of 3,5-Diiodo-L-thyronine (3,5-T2) versus its sulfate derivative:
Biological Activity | 3,5-Diiodo-L-thyronine (3,5-T2) | 3,5-Diiodo-L-thyronine 4'-O-Sulfate |
---|---|---|
Mitochondrial Oxidative Capacity | Increased | Enhanced |
Fatty Acid Oxidation | Increased | Enhanced |
Hepatic Steatosis Reduction | Yes | Yes |
Cold Tolerance Improvement | Yes | Yes |
Thyromimetic Activity | Moderate | Minimal |
Case Studies
- Animal Model Studies : In a study involving rats treated with 3,5-T2 sulfate, significant increases in mitochondrial function were observed. The treatment resulted in higher rates of fatty acid oxidation compared to controls .
- Human Serum Analysis : A feasibility study assessed endogenous levels of 3,5-T2 and its metabolites in human serum. The findings indicated that while 3,5-T2 had active roles in metabolism regulation, the sulfate form exhibited reduced intrinsic activity but could be reactivated under certain conditions .
Potential Therapeutic Applications
The unique properties of 3,5-Diiodo-L-thyronine 4'-O-sulfate suggest several potential therapeutic applications:
- Metabolic Disorders : Given its role in enhancing mitochondrial function and reducing hepatic fat accumulation, this compound may be explored as a treatment for obesity and non-alcoholic fatty liver disease (NAFLD) .
- Thyroid Dysfunction : The ability of 3,5-T2 sulfate to modulate thyroid hormone action could be beneficial in conditions like hypothyroidism or resistance to thyroid hormone .
Eigenschaften
IUPAC Name |
(2S)-2-amino-3-[3,5-diiodo-4-(4-sulfooxyphenoxy)phenyl]propanoic acid |
Source
|
---|---|---|
Source | PubChem | |
URL | https://pubchem.ncbi.nlm.nih.gov | |
Description | Data deposited in or computed by PubChem | |
InChI |
InChI=1S/C15H13I2NO7S/c16-11-5-8(7-13(18)15(19)20)6-12(17)14(11)24-9-1-3-10(4-2-9)25-26(21,22)23/h1-6,13H,7,18H2,(H,19,20)(H,21,22,23)/t13-/m0/s1 |
Source
|
Source | PubChem | |
URL | https://pubchem.ncbi.nlm.nih.gov | |
Description | Data deposited in or computed by PubChem | |
InChI Key |
HOUQDJLGYJSQKF-ZDUSSCGKSA-N |
Source
|
Source | PubChem | |
URL | https://pubchem.ncbi.nlm.nih.gov | |
Description | Data deposited in or computed by PubChem | |
Canonical SMILES |
C1=CC(=CC=C1OC2=C(C=C(C=C2I)CC(C(=O)O)N)I)OS(=O)(=O)O |
Source
|
Source | PubChem | |
URL | https://pubchem.ncbi.nlm.nih.gov | |
Description | Data deposited in or computed by PubChem | |
Isomeric SMILES |
C1=CC(=CC=C1OC2=C(C=C(C=C2I)C[C@@H](C(=O)O)N)I)OS(=O)(=O)O |
Source
|
Source | PubChem | |
URL | https://pubchem.ncbi.nlm.nih.gov | |
Description | Data deposited in or computed by PubChem | |
Molecular Formula |
C15H13I2NO7S |
Source
|
Source | PubChem | |
URL | https://pubchem.ncbi.nlm.nih.gov | |
Description | Data deposited in or computed by PubChem | |
Molecular Weight |
605.1 g/mol |
Source
|
Source | PubChem | |
URL | https://pubchem.ncbi.nlm.nih.gov | |
Description | Data deposited in or computed by PubChem | |
Haftungsausschluss und Informationen zu In-Vitro-Forschungsprodukten
Bitte beachten Sie, dass alle Artikel und Produktinformationen, die auf BenchChem präsentiert werden, ausschließlich zu Informationszwecken bestimmt sind. Die auf BenchChem zum Kauf angebotenen Produkte sind speziell für In-vitro-Studien konzipiert, die außerhalb lebender Organismen durchgeführt werden. In-vitro-Studien, abgeleitet von dem lateinischen Begriff "in Glas", beinhalten Experimente, die in kontrollierten Laborumgebungen unter Verwendung von Zellen oder Geweben durchgeführt werden. Es ist wichtig zu beachten, dass diese Produkte nicht als Arzneimittel oder Medikamente eingestuft sind und keine Zulassung der FDA für die Vorbeugung, Behandlung oder Heilung von medizinischen Zuständen, Beschwerden oder Krankheiten erhalten haben. Wir müssen betonen, dass jede Form der körperlichen Einführung dieser Produkte in Menschen oder Tiere gesetzlich strikt untersagt ist. Es ist unerlässlich, sich an diese Richtlinien zu halten, um die Einhaltung rechtlicher und ethischer Standards in Forschung und Experiment zu gewährleisten.