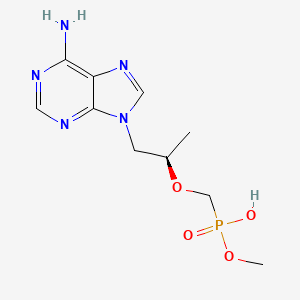
Tenofovir-Monomethylester
Übersicht
Beschreibung
Tenofovir Monomethyl Ester is an acyclic phosphonate nucleotide analogue and a reverse transcriptase inhibitor. It is primarily used as an anti-HIV agent and has shown significant antiviral activity against hepatitis B virus (HBV) as well . This compound is a derivative of tenofovir, which has been a cornerstone in antiretroviral therapy since its introduction .
Wissenschaftliche Forschungsanwendungen
Tenofovir Monomethyl Ester has a wide range of scientific research applications. In chemistry, it is used as a model compound to study nucleotide analogues. In biology and medicine, it is extensively researched for its antiviral properties, particularly against HIV and HBV . The compound has also been explored in nanomedicine, where it is combined with gold nanoparticles to enhance drug delivery and efficacy . Additionally, it has applications in studying the pharmacokinetics and pharmacodynamics of nucleotide analogues .
Wirkmechanismus
Target of Action
Tenofovir Monomethyl Ester, like its parent compound Tenofovir , primarily targets the reverse transcriptase enzyme . This enzyme plays a crucial role in the life cycle of retroviruses such as HIV, where it catalyzes the transcription of viral RNA into DNA, a necessary step for the virus to replicate within host cells .
Mode of Action
Tenofovir Monomethyl Ester is a nucleotide analog reverse transcriptase inhibitor (NtRTI) . It works by blocking the action of reverse transcriptase, thereby preventing the transcription of viral RNA into DNA . This effectively halts the replication of the virus, reducing viral load and slowing the progression of the disease .
Biochemical Pathways
Tenofovir Monomethyl Ester, as a nucleotide analog, integrates into the viral DNA chain during replication. By doing so, it causes premature termination of the DNA chain, effectively stopping the replication process . This disruption in the viral replication process leads to a decrease in viral load and a slowdown in the progression of the disease .
Pharmacokinetics
Tenofovir is known to diffuse widely, particularly in certain tissues such as the digestive tract, liver, and kidneys . .
Result of Action
The primary result of Tenofovir Monomethyl Ester’s action is a reduction in viral load, which slows the progression of diseases like HIV and Hepatitis B . By inhibiting the reverse transcriptase enzyme, it prevents the virus from replicating within host cells . This can lead to improved clinical outcomes for patients, including a slower progression of the disease and a reduction in the symptoms associated with these viral infections .
Action Environment
The action of Tenofovir Monomethyl Ester can be influenced by various environmental factors. For instance, the stability of Tenofovir prodrugs in intestinal fluid determines the actual amount of the prodrug at the absorption site, and hepatic/intestinal stability determines the maintenance amount of prodrug in circulation . Both of these factors influence the oral bioavailability of Tenofovir prodrugs
Biochemische Analyse
Biochemical Properties
Tenofovir Monomethyl Ester plays a significant role in biochemical reactions, particularly in the inhibition of viral replication . It interacts with various enzymes and proteins, including those involved in the replication of the Hepatitis B virus (HBV) . The nature of these interactions involves the compound binding to these biomolecules, thereby inhibiting their function and preventing the replication of the virus .
Cellular Effects
The effects of Tenofovir Monomethyl Ester on cells are profound. It influences cell function by impacting various cellular processes . For instance, it has been observed to have a correcting effect on disordered host hepatic biochemical metabolism, including the TCA cycle, glycolysis, pentose phosphate pathway, purine/pyrimidine metabolism, amino acid metabolism, ketone body metabolism, and phospholipid metabolism .
Molecular Mechanism
The molecular mechanism of action of Tenofovir Monomethyl Ester involves its binding interactions with biomolecules and its impact on gene expression . It exerts its effects at the molecular level by inhibiting enzymes involved in viral replication . This inhibition is achieved through the compound’s binding to these enzymes, which prevents them from functioning normally .
Temporal Effects in Laboratory Settings
In laboratory settings, the effects of Tenofovir Monomethyl Ester have been observed to change over time . It has been noted that the compound’s inhibition of HBV DNA replication is stronger after 9 days of treatment . Furthermore, the compound’s stability, degradation, and long-term effects on cellular function have been studied in both in vitro and in vivo studies .
Dosage Effects in Animal Models
The effects of Tenofovir Monomethyl Ester have been studied in animal models, with the compound exhibiting different effects at different dosages
Metabolic Pathways
Tenofovir Monomethyl Ester is involved in various metabolic pathways . It interacts with enzymes and cofactors within these pathways, potentially affecting metabolic flux or metabolite levels .
Transport and Distribution
The transport and distribution of Tenofovir Monomethyl Ester within cells and tissues are critical aspects of its function
Vorbereitungsmethoden
The synthesis of Tenofovir Monomethyl Ester involves several key steps. One common synthetic route starts with the acyclic precursor diaminomalononitrile. This precursor undergoes a four-step protocol to produce tenofovir, which is then esterified to form Tenofovir Monomethyl Ester . The key transformations include aminolysis-hydrolysis, alkylation, and cleavage of the phosphonate ester . Industrial production methods often involve optimizing these steps to improve yield and reduce costs .
Analyse Chemischer Reaktionen
Tenofovir Monomethyl Ester undergoes various chemical reactions, including oxidation, reduction, and substitution. Common reagents used in these reactions include tosylated diethyl(hydroxymethyl) phosphonate and bromotrimethylsilane . The major products formed from these reactions are typically intermediates that lead to the active form of tenofovir .
Vergleich Mit ähnlichen Verbindungen
Tenofovir Monomethyl Ester is often compared with other tenofovir prodrugs such as tenofovir disoproxil fumarate and tenofovir alafenamide . While all these compounds share a similar mechanism of action, Tenofovir Monomethyl Ester is unique in its ester form, which affects its pharmacokinetic properties . Tenofovir alafenamide, for example, has better stability and bioavailability compared to tenofovir disoproxil fumarate . Other similar compounds include tenofovir amibufenamide fumarate, which also shows strong antiviral activity .
Eigenschaften
IUPAC Name |
[(2R)-1-(6-aminopurin-9-yl)propan-2-yl]oxymethyl-methoxyphosphinic acid | |
---|---|---|
Source | PubChem | |
URL | https://pubchem.ncbi.nlm.nih.gov | |
Description | Data deposited in or computed by PubChem | |
InChI |
InChI=1S/C10H16N5O4P/c1-7(19-6-20(16,17)18-2)3-15-5-14-8-9(11)12-4-13-10(8)15/h4-5,7H,3,6H2,1-2H3,(H,16,17)(H2,11,12,13)/t7-/m1/s1 | |
Source | PubChem | |
URL | https://pubchem.ncbi.nlm.nih.gov | |
Description | Data deposited in or computed by PubChem | |
InChI Key |
QNIIFMBLEIMHIO-SSDOTTSWSA-N | |
Source | PubChem | |
URL | https://pubchem.ncbi.nlm.nih.gov | |
Description | Data deposited in or computed by PubChem | |
Canonical SMILES |
CC(CN1C=NC2=C(N=CN=C21)N)OCP(=O)(O)OC | |
Source | PubChem | |
URL | https://pubchem.ncbi.nlm.nih.gov | |
Description | Data deposited in or computed by PubChem | |
Isomeric SMILES |
C[C@H](CN1C=NC2=C(N=CN=C21)N)OCP(=O)(O)OC | |
Source | PubChem | |
URL | https://pubchem.ncbi.nlm.nih.gov | |
Description | Data deposited in or computed by PubChem | |
Molecular Formula |
C10H16N5O4P | |
Source | PubChem | |
URL | https://pubchem.ncbi.nlm.nih.gov | |
Description | Data deposited in or computed by PubChem | |
DSSTOX Substance ID |
DTXSID00858427 | |
Record name | Methyl hydrogen ({[(2R)-1-(6-amino-9H-purin-9-yl)propan-2-yl]oxy}methyl)phosphonate | |
Source | EPA DSSTox | |
URL | https://comptox.epa.gov/dashboard/DTXSID00858427 | |
Description | DSSTox provides a high quality public chemistry resource for supporting improved predictive toxicology. | |
Molecular Weight |
301.24 g/mol | |
Source | PubChem | |
URL | https://pubchem.ncbi.nlm.nih.gov | |
Description | Data deposited in or computed by PubChem | |
CAS No. |
123155-85-1 | |
Record name | Methyl hydrogen ({[(2R)-1-(6-amino-9H-purin-9-yl)propan-2-yl]oxy}methyl)phosphonate | |
Source | EPA DSSTox | |
URL | https://comptox.epa.gov/dashboard/DTXSID00858427 | |
Description | DSSTox provides a high quality public chemistry resource for supporting improved predictive toxicology. | |
Haftungsausschluss und Informationen zu In-Vitro-Forschungsprodukten
Bitte beachten Sie, dass alle Artikel und Produktinformationen, die auf BenchChem präsentiert werden, ausschließlich zu Informationszwecken bestimmt sind. Die auf BenchChem zum Kauf angebotenen Produkte sind speziell für In-vitro-Studien konzipiert, die außerhalb lebender Organismen durchgeführt werden. In-vitro-Studien, abgeleitet von dem lateinischen Begriff "in Glas", beinhalten Experimente, die in kontrollierten Laborumgebungen unter Verwendung von Zellen oder Geweben durchgeführt werden. Es ist wichtig zu beachten, dass diese Produkte nicht als Arzneimittel oder Medikamente eingestuft sind und keine Zulassung der FDA für die Vorbeugung, Behandlung oder Heilung von medizinischen Zuständen, Beschwerden oder Krankheiten erhalten haben. Wir müssen betonen, dass jede Form der körperlichen Einführung dieser Produkte in Menschen oder Tiere gesetzlich strikt untersagt ist. Es ist unerlässlich, sich an diese Richtlinien zu halten, um die Einhaltung rechtlicher und ethischer Standards in Forschung und Experiment zu gewährleisten.