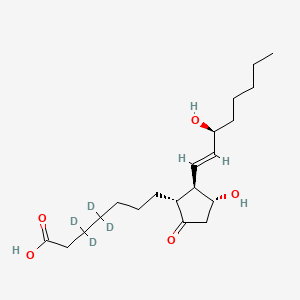
Prostaglandin E1-d4
Übersicht
Beschreibung
Prostaglandin E1-d4 is a deuterium-labeled analog of Prostaglandin E1, also known as Alprostadil. Prostaglandin E1 is a naturally occurring prostaglandin used in medicine for its vasodilatory properties, which help in treating conditions like erectile dysfunction and peripheral vascular diseases. The deuterium labeling in this compound enhances its stability and allows for its use in various scientific research applications .
Wirkmechanismus
- These receptors are involved in diverse biological processes, including blood pressure regulation, inflammation, pain perception, and cell survival .
- In neonates with ductal-dependent lesions, PGE1 prevents or reverses the functional closure of the ductus arteriosus (DA) by relaxing DA smooth muscle .
Target of Action
Mode of Action
Biochemical Pathways
Biochemische Analyse
Biochemical Properties
Prostaglandin E1-d4 interacts with various enzymes and proteins. It is an analogue of arachidonic acid (AA), a polyunsaturated fatty acid found in membrane phospholipids . This compound is known to interact with mouse EP3, EP4, EP2, IP, and EP1 receptors .
Cellular Effects
This compound has significant effects on various types of cells and cellular processes. It induces vasodilation and inhibits platelet aggregation . In high glucose-treated HK-2 proximal tubular cells, this compound significantly enhanced cell viability and suppressed the c-Jun N-terminal kinase (JNK) and the mitochondrial apoptosis-related protein expressions .
Molecular Mechanism
The molecular mechanism of this compound involves its binding interactions with biomolecules, enzyme inhibition or activation, and changes in gene expression. It blocks the activation of the JNK/Bim pathway in HK-2 cells, with JNK being an upstream regulator of Bim .
Temporal Effects in Laboratory Settings
In laboratory settings, this compound has shown to reduce ventricular hypertrophy, protein expressions of endothelin-1 (ET-1) and endothelin receptor A (ERA), and the expression of fibrosis over time .
Dosage Effects in Animal Models
In animal models, the effects of this compound vary with different dosages. For instance, in a study involving uninephrectomized streptozotocin-induced diabetic rats, the rats were administered this compound (10 μg· kg−1· d−1, iv.) for 10 consecutive days .
Metabolic Pathways
This compound is involved in the metabolic pathways that include interactions with enzymes or cofactors. It derives from dihomogammalinolenic acid (20:3), being an analogue of arachidonic acid (AA) .
Transport and Distribution
It is known that this compound accumulates in sites of inflammation or vascular lesions .
Subcellular Localization
The subcellular localization of this compound is not explicitly known. Related compounds such as Prostaglandin Endoperoxide H Synthases-1 and -2 were found on the lumenal surfaces of the endoplasmic reticulum (ER) and nuclear envelope of human monocytes . This might provide some insights into the potential subcellular localization of this compound.
Vorbereitungsmethoden
Synthesewege und Reaktionsbedingungen: Die Synthese von Prostaglandin E1-d4 beinhaltet die Einarbeitung von Deuteriumatomen in das Prostaglandin E1-Molekül. Eine gängige Methode ist die Verwendung deuterierter Reagenzien im Syntheseprozess. So können beispielsweise deuterierte Lösungsmittel und Katalysatoren eingesetzt werden, um Wasserstoffatome an bestimmten Positionen des Moleküls durch Deuterium zu ersetzen .
Industrielle Produktionsmethoden: Die industrielle Produktion von this compound erfolgt typischerweise in großtechnischer Synthese unter Verwendung deuterierter Ausgangsmaterialien. Der Prozess kann Schritte wie:
- Lösen von Prostaglandin E1 in einer Lösung aus Lactose und tert-Butanol.
- Einstellen und Halten des pH-Wertes mit einem organischen Säurepuffer.
- Einfrieren und Trocknen der Formulierung, um ein stabiles Produkt mit niedrigem Feuchtigkeitsgehalt zu erhalten .
Analyse Chemischer Reaktionen
Arten von Reaktionen: Prostaglandin E1-d4 unterliegt verschiedenen chemischen Reaktionen, darunter:
Oxidation: Diese Reaktion kann zur Bildung verschiedener oxidierter Derivate führen.
Reduktion: Reduktionsreaktionen können die funktionellen Gruppen innerhalb des Moleküls verändern.
Substitution: Deuteriumatome können anstelle von Wasserstoffatomen substituiert werden, um markierte Analoga zu erzeugen.
Häufige Reagenzien und Bedingungen:
Oxidation: Häufige Oxidationsmittel sind Kaliumpermanganat und Chromtrioxid.
Reduktion: Reduktionsmittel wie Lithiumaluminiumhydrid und Natriumborhydrid werden häufig verwendet.
Substitution: Deuterierte Reagenzien wie Deuteriumoxid und deuterierte Lösungsmittel werden eingesetzt.
Hauptprodukte: Die Hauptprodukte, die aus diesen Reaktionen gebildet werden, sind verschiedene deuteriummarkierte Derivate von Prostaglandin E1, die für Forschungs- und analytische Zwecke verwendet werden .
Wissenschaftliche Forschungsanwendungen
Prostaglandin E1-d4 hat eine breite Palette von Anwendungen in der wissenschaftlichen Forschung, darunter:
Chemie: Als Tracer in der Massenspektrometrie und anderen analytischen Techniken eingesetzt, um Stoffwechselwege und den Arzneimittelstoffwechsel zu untersuchen.
Biologie: In Studien zur Zellsignalisierung und Rezeptorinteraktionen eingesetzt.
Medizin: Für seine potenziellen therapeutischen Wirkungen bei der Behandlung von Herz-Kreislauf-Erkrankungen und erektiler Dysfunktion untersucht.
Industrie: In der Entwicklung neuer Arzneimittel und als Referenzstandard in der Qualitätskontrolle eingesetzt
5. Wirkmechanismus
This compound übt seine Wirkungen aus, indem es an spezifische Prostaglandinrezeptoren bindet, wie z. B. EP1, EP2, EP3 und EP4. Diese Rezeptoren sind G-Protein-gekoppelte Rezeptoren, die verschiedene physiologische Reaktionen vermitteln, darunter Vasodilatation, Hemmung der Thrombozytenaggregation und Modulation von Entzündungen. Die Bindung von this compound an diese Rezeptoren aktiviert intrazelluläre Signalwege, die zu den beobachteten biologischen Wirkungen führen .
Ähnliche Verbindungen:
Prostaglandin E2: Ähnlich in der Struktur, hat aber unterschiedliche Rezeptoraffinitäten und physiologische Wirkungen.
Prostaglandin F2α: Bekannt für seine Rolle bei der Kontraktion der glatten Muskulatur und verschiedene therapeutische Anwendungen.
Prostaglandin I2 (Prostacyclin): Hauptsächlich an der Vasodilatation und der Hemmung der Thrombozytenaggregation beteiligt
Einzigartigkeit: this compound ist aufgrund seiner Deuteriummarkierung einzigartig, die seine Stabilität erhöht und präzise analytische Studien ermöglicht. Diese Markierung macht es besonders wertvoll in Forschungsumgebungen, in denen eine genaue Quantifizierung und Verfolgung von Stoffwechselwegen erforderlich sind .
Vergleich Mit ähnlichen Verbindungen
Prostaglandin E2: Similar in structure but has different receptor affinities and physiological effects.
Prostaglandin F2α: Known for its role in smooth muscle contraction and different therapeutic applications.
Prostaglandin I2 (Prostacyclin): Primarily involved in vasodilation and inhibition of platelet aggregation
Uniqueness: Prostaglandin E1-d4 is unique due to its deuterium labeling, which enhances its stability and allows for precise analytical studies. This labeling makes it particularly valuable in research settings where accurate quantification and tracing of metabolic pathways are required .
Biologische Aktivität
Prostaglandin E1-d4 (PGE1-d4) is a stable isotope-labeled analog of Prostaglandin E1 (PGE1), which has garnered attention for its diverse biological activities. This article explores the biological mechanisms, therapeutic applications, and research findings related to PGE1-d4, highlighting its efficacy in various medical contexts.
Overview of this compound
PGE1-d4 is a synthetic derivative of PGE1, which is known for its vasodilatory effects and role in maintaining ductal patency in neonates with congenital heart defects. The introduction of deuterium in PGE1-d4 enhances its metabolic stability, potentially leading to prolonged biological activity compared to its non-labeled counterpart.
PGE1-d4 exerts its biological effects through interaction with specific receptors, primarily the prostaglandin E-type receptors (EP1-4) and the prostaglandin I-type receptor (IP). These receptors are widely distributed across various tissues and organs, mediating numerous physiological responses:
- Vasodilation : PGE1-d4 promotes vascular smooth muscle relaxation, leading to increased blood flow and reduced peripheral resistance.
- Inhibition of Platelet Aggregation : PGE1-d4 inhibits platelet activation and aggregation through binding to IP and EP3 receptors, which is crucial for preventing thrombosis.
- Pain Modulation : It has been shown to suppress acute pain responses, possibly by modulating inflammatory pathways.
1. Cardiovascular Applications
PGE1-d4 is particularly significant in neonatal care for maintaining ductal patency in cases of ductal-dependent congenital heart diseases. Its administration can facilitate adequate systemic and pulmonary blood flow until surgical interventions can be performed.
Study | Population | Outcome |
---|---|---|
Leoni et al. (1984) | Neonates with ductal-dependent lesions | Improved ductal patency and reduced mortality rates |
Meckler et al. (2009) | Patients undergoing cardiac surgery | Enhanced systemic circulation pre-surgery |
2. Chronic Myelogenous Leukemia (CML)
Research indicates that PGE1-d4 may target leukemic stem cells (LSCs) effectively, inhibiting their self-renewal capabilities. In a preclinical murine model, PGE1-d4 was shown to impair LSC persistence without significantly affecting normal hematopoiesis.
Study | Model | Findings |
---|---|---|
Faye-Peterson et al. (2017) | Murine CML model | Reduced LSC activity via EP4 receptor modulation |
3. Pain Management
PGE1-d4's analgesic properties have been observed in various animal models. For instance, it significantly reduced pain response in formalin-induced pain models, suggesting potential applications in pain management therapies.
Case Study 1: Use in Neonates
A retrospective analysis of neonates treated with PGE1-d4 for maintaining ductal patency showed a significant reduction in mortality associated with congenital heart defects. The study highlighted the importance of timely intervention using PGE1-d4 to stabilize hemodynamic status prior to surgical correction.
Case Study 2: Chronic Pain Management
In a clinical trial assessing the efficacy of PGE1-d4 for chronic pain relief, patients reported a marked decrease in pain levels when administered the compound compared to placebo controls. This suggests that PGE1-d4 could serve as an adjunct therapy for chronic pain conditions.
Research Findings
Recent studies have elucidated various aspects of PGE1-d4's biological activity:
- Vascular Permeability : In vivo studies demonstrated that PGE1-d4 significantly increases vascular permeability, enhancing drug delivery systems in targeted therapies.
- Anti-inflammatory Effects : PGE1-d4 modulates inflammatory responses by balancing pro-inflammatory and anti-inflammatory factors, making it a candidate for treating inflammatory diseases.
Eigenschaften
IUPAC Name |
3,3,4,4-tetradeuterio-7-[(1R,2R,3R)-3-hydroxy-2-[(E,3S)-3-hydroxyoct-1-enyl]-5-oxocyclopentyl]heptanoic acid | |
---|---|---|
Source | PubChem | |
URL | https://pubchem.ncbi.nlm.nih.gov | |
Description | Data deposited in or computed by PubChem | |
InChI |
InChI=1S/C20H34O5/c1-2-3-6-9-15(21)12-13-17-16(18(22)14-19(17)23)10-7-4-5-8-11-20(24)25/h12-13,15-17,19,21,23H,2-11,14H2,1H3,(H,24,25)/b13-12+/t15-,16+,17+,19+/m0/s1/i5D2,8D2 | |
Source | PubChem | |
URL | https://pubchem.ncbi.nlm.nih.gov | |
Description | Data deposited in or computed by PubChem | |
InChI Key |
GMVPRGQOIOIIMI-HKEIXBCBSA-N | |
Source | PubChem | |
URL | https://pubchem.ncbi.nlm.nih.gov | |
Description | Data deposited in or computed by PubChem | |
Canonical SMILES |
CCCCCC(C=CC1C(CC(=O)C1CCCCCCC(=O)O)O)O | |
Source | PubChem | |
URL | https://pubchem.ncbi.nlm.nih.gov | |
Description | Data deposited in or computed by PubChem | |
Isomeric SMILES |
[2H]C([2H])(CCC[C@@H]1[C@H]([C@@H](CC1=O)O)/C=C/[C@H](CCCCC)O)C([2H])([2H])CC(=O)O | |
Source | PubChem | |
URL | https://pubchem.ncbi.nlm.nih.gov | |
Description | Data deposited in or computed by PubChem | |
Molecular Formula |
C20H34O5 | |
Source | PubChem | |
URL | https://pubchem.ncbi.nlm.nih.gov | |
Description | Data deposited in or computed by PubChem | |
Molecular Weight |
358.5 g/mol | |
Source | PubChem | |
URL | https://pubchem.ncbi.nlm.nih.gov | |
Description | Data deposited in or computed by PubChem | |
Haftungsausschluss und Informationen zu In-Vitro-Forschungsprodukten
Bitte beachten Sie, dass alle Artikel und Produktinformationen, die auf BenchChem präsentiert werden, ausschließlich zu Informationszwecken bestimmt sind. Die auf BenchChem zum Kauf angebotenen Produkte sind speziell für In-vitro-Studien konzipiert, die außerhalb lebender Organismen durchgeführt werden. In-vitro-Studien, abgeleitet von dem lateinischen Begriff "in Glas", beinhalten Experimente, die in kontrollierten Laborumgebungen unter Verwendung von Zellen oder Geweben durchgeführt werden. Es ist wichtig zu beachten, dass diese Produkte nicht als Arzneimittel oder Medikamente eingestuft sind und keine Zulassung der FDA für die Vorbeugung, Behandlung oder Heilung von medizinischen Zuständen, Beschwerden oder Krankheiten erhalten haben. Wir müssen betonen, dass jede Form der körperlichen Einführung dieser Produkte in Menschen oder Tiere gesetzlich strikt untersagt ist. Es ist unerlässlich, sich an diese Richtlinien zu halten, um die Einhaltung rechtlicher und ethischer Standards in Forschung und Experiment zu gewährleisten.