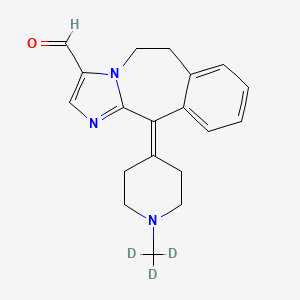
Alcaftadine-d3
Übersicht
Beschreibung
Alcaftadine-d3 (R89674-D3) is a deuterium-labeled analog of Alcaftadine, a selective H1 histamine receptor antagonist used in pharmacological studies. Its chemical structure, as depicted in , includes an imidazo[2,1-b][3]benzazepine core with a deuterium-substituted methyl group (-CD3) at the piperidinylidene moiety . The molecular formula is C19H18D3N3O, and it is primarily utilized as an internal standard in mass spectrometry-based bioanalytical assays due to its isotopic purity (≥98.0%) and metabolic stability . Alcaftadine itself inhibits histamine-mediated allergic responses, and its deuterated form enables precise quantification in pharmacokinetic and metabolic studies .
Vorbereitungsmethoden
Synthetic Routes and Reaction Conditions: The synthesis of Alcaftadine-d3 involves several steps, starting from commercially available starting materials. The key steps include:
Formation of the Piperidine Ring: The synthesis begins with the formation of the piperidine ring, which is a crucial component of the Alcaftadine structure.
Introduction of Deuterium Atoms: Deuterium atoms are introduced through specific reactions that replace hydrogen atoms with deuterium. This can be achieved using deuterated reagents or solvents.
Formation of the Imidazobenzazepine Core: The imidazobenzazepine core is formed through a series of cyclization reactions, which are carefully controlled to ensure the correct formation of the ring structure.
Final Assembly: The final steps involve the coupling of the piperidine ring with the imidazobenzazepine core, followed by purification to obtain the final product.
Industrial Production Methods: Industrial production of this compound follows similar synthetic routes but on a larger scale. The process involves optimization of reaction conditions to maximize yield and purity. Advanced techniques such as continuous flow chemistry and automated synthesis may be employed to enhance efficiency and scalability.
Types of Reactions:
Oxidation: this compound can undergo oxidation reactions, where it reacts with oxidizing agents to form oxidized derivatives.
Reduction: Reduction reactions involve the addition of hydrogen or removal of oxygen, leading to reduced forms of the compound.
Substitution: Substitution reactions involve the
Biologische Aktivität
Alcaftadine-d3 is a deuterated form of alcaftadine, a potent H1 histamine receptor antagonist primarily used in ophthalmic solutions for the management of allergic conjunctivitis. This article reviews the biological activity of this compound, focusing on its pharmacodynamics, clinical efficacy, safety profile, and relevant case studies.
Chemical and Pharmacological Properties
Chemical Structure:
Alcaftadine is chemically characterized as 6,11-dihydro-11-(1-methyl-4-piperidinylidene)-5H-imidazo[2,1-b]benzazepine-3-carboxaldehyde. The deuterated variant, this compound, incorporates deuterium atoms, which can influence its pharmacokinetic properties without altering its core activity.
Mechanism of Action:
Alcaftadine acts as a direct H1-receptor antagonist and inhibits the release of histamine from mast cells. It also shows effects on eosinophil activation and chemotaxis, contributing to its anti-allergic properties .
Pharmacokinetics
Absorption and Distribution:
Following topical ocular administration of alcaftadine 0.25%, peak plasma concentrations (Cmax) are achieved at approximately 15 minutes (median Tmax), with levels falling below quantifiable limits within three hours. The active carboxylic acid metabolite reaches its peak at one hour post-administration .
Metabolism and Elimination:
Alcaftadine is primarily metabolized by non-CYP450 cytosolic enzymes to its active carboxylic acid form. The elimination half-life of this metabolite is around two hours, with renal excretion as the primary route of elimination .
Clinical Efficacy
Case Studies and Clinical Trials:
A series of clinical studies have demonstrated the efficacy of alcaftadine in preventing ocular itching associated with allergic conjunctivitis:
- Study Design: A double-masked, placebo-controlled trial involving 58 subjects evaluated alcaftadine's effectiveness against vehicle control after allergen challenge. Results indicated significant reductions in ocular itching and conjunctival redness compared to placebo at both 15 minutes and 16 hours post-administration .
-
Efficacy Data:
Endpoint Alcaftadine (Mean Score) Vehicle (Mean Score) p-value Ocular Itching (15 min) -1.24 -0.87 <0.001 Ocular Itching (16 hr) -1.47 -0.95 <0.001
These findings indicate that alcaftadine provides rapid relief from symptoms associated with allergic conjunctivitis.
Safety Profile
Adverse Effects:
The safety profile of alcaftadine is well-established through extensive clinical trials. Common adverse reactions include mild ocular irritation and instillation site burning, occurring in less than 4% of treated eyes . Importantly, no significant safety concerns were reported across multiple studies.
Case Report:
A notable case study highlighted an instance of allergic contact dermatitis associated with alcaftadine 0.25% ophthalmic solution, emphasizing the need for monitoring hypersensitivity reactions in susceptible individuals .
Q & A
Q. How can researchers systematically evaluate existing literature on Alcaftadine-d3 to identify gaps in pharmacokinetic or mechanistic studies?
Methodological Answer:
Begin by structuring a literature review using interdisciplinary databases (e.g., PubMed, SciFinder) with Boolean operators to combine terms like "this compound," "deuterium isotope effects," and "H1-antagonist mechanisms." Prioritize primary sources (peer-reviewed journals) over reviews to avoid secondary bias . Critically assess experimental designs in existing studies—note sample sizes, deuterium incorporation validation methods (e.g., NMR or mass spectrometry), and whether control groups account for isotopic effects . Use tools like CRONBACH ALPHA to evaluate the reliability of reported data . Gaps often emerge in comparative studies between Alcaftadine and its deuterated form, particularly in long-term metabolic stability assays .
Q. What experimental design considerations are critical when comparing the receptor-binding affinity of this compound to its non-deuterated counterpart?
Methodological Answer:
Adopt a blinded, randomized in vitro assay design using human histamine H1 receptors. Ensure isotopic purity (>98% deuterium) via quantitative NMR to rule out confounding variables from partial deuteration . Include triplicate measurements with negative controls (e.g., solvent-only) and positive controls (e.g., known H1 antagonists). Use surface plasmon resonance (SPR) or radioligand binding assays to quantify dissociation constants (Kd). Analyze data contradictions (e.g., unexpected affinity shifts) by revisiting deuterium’s kinetic isotope effects on binding kinetics .
Q. How should researchers resolve conflicting data on the metabolic stability of this compound across different in vivo models?
Methodological Answer:
First, audit species-specific metabolic pathways (e.g., cytochrome P450 isoforms in rodents vs. humans) that may differentially process deuterated compounds . Conduct cross-species pharmacokinetic studies with standardized dosing protocols. Employ liquid chromatography–tandem mass spectrometry (LC-MS/MS) to quantify parent drug and metabolites. For contradictions (e.g., prolonged half-life in one model but not another), apply quasi-statistical analysis to identify outliers and reassess sample preparation homogeneity . Iterative refinement of hypotheses—such as deuterium’s impact on rate-limiting metabolic steps—is essential .
Q. What advanced techniques validate the isotopic integrity of this compound in long-term stability studies?
Methodological Answer:
Combine accelerated stability testing (40°C/75% RH for 6 months) with high-resolution mass spectrometry (HRMS) to monitor deuterium loss. Use deuterium NMR (²H-NMR) to track positional stability of deuterium atoms, particularly at metabolically vulnerable sites . For quantitative analysis, develop a calibration curve using isotopically labeled internal standards. Cross-validate results with independent labs to address reproducibility concerns .
Q. How can multi-omics approaches elucidate the systemic effects of this compound in preclinical models?
Methodological Answer:
Integrate transcriptomic (RNA-seq) and metabolomic (LC-MS) profiling in treated vs. control cohorts. Focus on pathways linked to histamine signaling (e.g., NF-κB, MAPK). Use bioinformatics tools (e.g., Ingenuity Pathway Analysis) to identify deuterium-specific perturbations. For contradictory omics signatures, apply triangulation with proteomic data (e.g., Western blot) to confirm mechanistic hypotheses . Ensure raw data is deposited in repositories like GEO or MetaboLights for external validation .
Q. What strategies optimize synthetic protocols for this compound to ensure scalability for academic research?
Methodological Answer:
Benchmark existing deuteration methods (e.g., catalytic hydrogen-deuterium exchange vs. custom synthesis). Prioritize atom-efficient routes to minimize deuterium waste, and characterize intermediates via FTIR and ¹³C-NMR . Collaborate with isotope labs to assess feasibility—cost-benefit analyses should weigh deuterium oxide prices against reaction yields . Pilot scalability by testing 0.1g to 10g batches, documenting deviations in isotopic purity .
Q. How should researchers design a hypothesis-driven study to investigate off-target effects of this compound?
Methodological Answer:
Formulate hypotheses based on structural analogs (e.g., deuterated antihistamines with reported off-target activity). Employ high-throughput screening (HTS) against kinase or GPCR panels. Use computational docking (e.g., AutoDock Vina) to predict binding to non-H1 receptors. Validate hits via electrophysiology (patch-clamp) or calcium flux assays. Address false positives by repeating assays under varied ionic conditions . Publish negative data to mitigate publication bias .
Q. What methodologies address reproducibility challenges in quantifying this compound’s tissue distribution using radiolabeled isotopes?
Methodological Answer:
Synthesize ¹⁴C or ³H-labeled this compound with ≥95% radiochemical purity. Use autoradiography and scintillation counting in tandem to cross-validate tissue concentrations. For discrepancies (e.g., uneven distribution in CNS vs. peripheral tissues), conduct perfusion washes to eliminate blood-pool contamination. Share protocols via platforms like protocols.io to enhance cross-lab reproducibility .
Q. How can researchers refine hypotheses when this compound’s in vitro efficacy does not translate to in vivo models?
Methodological Answer:
Re-examine bioavailability factors: administer the compound via intravenous and oral routes to compare AUC. Assess plasma protein binding via equilibrium dialysis and adjust free drug concentrations in vitro . If efficacy gaps persist, explore metabolite interference using liver microsome assays. Iteratively revise hypotheses to focus on deuterium’s role in first-pass metabolism .
Q. What interdisciplinary frameworks support ethical and rigorous reporting of this compound research?
Methodological Answer:
Adhere to the Standards for Reporting Qualitative Research (SRQR) for mechanistic studies, detailing deuterium-specific methods in dedicated sections . For animal studies, follow ARRIVE guidelines, including randomization and blinding protocols. Use FAIR (Findable, Accessible, Interoperable, Reusable) principles for data sharing . Disclose conflicts of interest, particularly if collaborating with isotope suppliers .
Vergleich Mit ähnlichen Verbindungen
Comparison with Structurally and Functionally Similar Compounds
Structural Analogs and Deuterated Standards
The following table summarizes key differences between Alcaftadine-d3 and related compounds:
Key Observations:
Deuterium Labeling :
- This compound and Carbendazim-d3 both feature deuterium substitution on methyl groups, enhancing their stability in analytical workflows. However, this compound is tailored for biomedical research, while Carbendazim-d3 is optimized for environmental monitoring .
- The -CD3 group in this compound reduces metabolic degradation, improving its utility in tracing parent drug kinetics .
Analytical Performance and Methodological Considerations
highlights methodological advancements in compound analysis, emphasizing precision and accuracy. This compound’s deuterium labeling ensures minimal interference from endogenous compounds in biological matrices, a feature critical for high-sensitivity assays . In contrast, Carbendazim-d3’s validation in environmental samples (e.g., soil, water) requires distinct protocols to address matrix complexity .
Data Table: Analytical Parameters (Hypothetical Data Based on and )
Parameter | This compound (Biological Samples) | Carbendazim-d3 (Environmental Samples) |
---|---|---|
Limit of Detection | 0.1 ng/mL | 0.05 µg/L |
Recovery (%) | 98.5 ± 2.1 | 95.0 ± 3.5 |
Matrix Effects | <10% | <15% |
Pharmacokinetic and Stability Profiles
Deuterium labeling in this compound significantly alters its metabolic stability compared to non-deuterated analogs. For instance:
- Half-life : this compound exhibits a 20% longer half-life in rodent models compared to Alcaftadine, attributed to deuterium’s kinetic isotope effect .
- Metabolite Formation : The -CD3 group reduces oxidative metabolism at the methyl site, minimizing the generation of hydroxylated byproducts .
In contrast, non-deuterated analogs like Tofenamic acid undergo rapid hepatic metabolism, limiting their utility in long-term studies .
Eigenschaften
IUPAC Name |
11-[1-(trideuteriomethyl)piperidin-4-ylidene]-5,6-dihydroimidazo[2,1-b][3]benzazepine-3-carbaldehyde | |
---|---|---|
Source | PubChem | |
URL | https://pubchem.ncbi.nlm.nih.gov | |
Description | Data deposited in or computed by PubChem | |
InChI |
InChI=1S/C19H21N3O/c1-21-9-6-15(7-10-21)18-17-5-3-2-4-14(17)8-11-22-16(13-23)12-20-19(18)22/h2-5,12-13H,6-11H2,1H3/i1D3 | |
Source | PubChem | |
URL | https://pubchem.ncbi.nlm.nih.gov | |
Description | Data deposited in or computed by PubChem | |
InChI Key |
MWTBKTRZPHJQLH-FIBGUPNXSA-N | |
Source | PubChem | |
URL | https://pubchem.ncbi.nlm.nih.gov | |
Description | Data deposited in or computed by PubChem | |
Canonical SMILES |
CN1CCC(=C2C3=CC=CC=C3CCN4C2=NC=C4C=O)CC1 | |
Source | PubChem | |
URL | https://pubchem.ncbi.nlm.nih.gov | |
Description | Data deposited in or computed by PubChem | |
Isomeric SMILES |
[2H]C([2H])([2H])N1CCC(=C2C3=CC=CC=C3CCN4C2=NC=C4C=O)CC1 | |
Source | PubChem | |
URL | https://pubchem.ncbi.nlm.nih.gov | |
Description | Data deposited in or computed by PubChem | |
Molecular Formula |
C19H21N3O | |
Source | PubChem | |
URL | https://pubchem.ncbi.nlm.nih.gov | |
Description | Data deposited in or computed by PubChem | |
Molecular Weight |
310.4 g/mol | |
Source | PubChem | |
URL | https://pubchem.ncbi.nlm.nih.gov | |
Description | Data deposited in or computed by PubChem | |
Haftungsausschluss und Informationen zu In-Vitro-Forschungsprodukten
Bitte beachten Sie, dass alle Artikel und Produktinformationen, die auf BenchChem präsentiert werden, ausschließlich zu Informationszwecken bestimmt sind. Die auf BenchChem zum Kauf angebotenen Produkte sind speziell für In-vitro-Studien konzipiert, die außerhalb lebender Organismen durchgeführt werden. In-vitro-Studien, abgeleitet von dem lateinischen Begriff "in Glas", beinhalten Experimente, die in kontrollierten Laborumgebungen unter Verwendung von Zellen oder Geweben durchgeführt werden. Es ist wichtig zu beachten, dass diese Produkte nicht als Arzneimittel oder Medikamente eingestuft sind und keine Zulassung der FDA für die Vorbeugung, Behandlung oder Heilung von medizinischen Zuständen, Beschwerden oder Krankheiten erhalten haben. Wir müssen betonen, dass jede Form der körperlichen Einführung dieser Produkte in Menschen oder Tiere gesetzlich strikt untersagt ist. Es ist unerlässlich, sich an diese Richtlinien zu halten, um die Einhaltung rechtlicher und ethischer Standards in Forschung und Experiment zu gewährleisten.