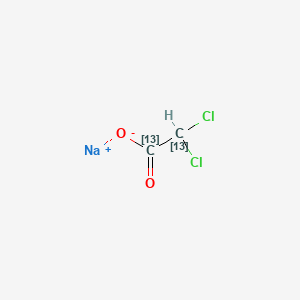
Sodium dichloroacetate-13C2
Übersicht
Beschreibung
Sodium dichloroacetate-13C2, also known as Dichloroacetic acid-13C2 sodium salt, is a compound with a molecular weight of 152.91 . It is used in metabolomics and MS/MS standards .
Synthesis Analysis
Sodium dichloroacetate-13C2 is synthesized in laboratories . It has a linear formula of Cl213CH13CO2Na . The introduction of a dichloroacetic acid fragment into a molecule is an approach to anticancer prodrug development .Molecular Structure Analysis
The molecular formula of Sodium dichloroacetate-13C2 is 13C2Cl2NaO2 . The SMILES string representation is [Na+].[O-]13C13CHCl .Chemical Reactions Analysis
Sodium dichloroacetate-13C2 reactivates oxidative phosphorylation in cancer cell mitochondria, reducing apoptosis resistance in cancer cells . It also inhibits the proliferation of tumor cells and CD133+ cancer-stem-like cells .Physical And Chemical Properties Analysis
Sodium dichloroacetate-13C2 is a powder with a melting point of 198 °C (dec.) . It should be stored at room temperature away from light and moisture .Wissenschaftliche Forschungsanwendungen
Anti-Cancer Effects
Sodium dichloroacetate (DCA) has been reported to have anti-cancer effects by reversing tumor-associated glycolysis . This is particularly relevant in the context of lung cancer, where DCA has been shown to cause a concentration- and time-dependent decrease in the viability and colony growth of non-small cell lung cancer (NSCLC) cell lines .
Combination Therapies for Cancer
DCA has been studied for its potential to enhance the anti-cancer effect of other therapies. For instance, it has been found to significantly enhance the anti-cancer effect of cisplatin in LNM35, a type of NSCLC . Additionally, the combination of DCA with gefitinib or erlotinib leads to additive effects on the inhibition of LNM35 colony growth .
Treatment of Hypoglycemia-Induced Injury
DCA has been studied for its effects on mitochondrial dysfunction and neuronal death following hypoglycemia-induced injury . It has been found that DCA treatment can reduce neuronal death through the improvement of glycolysis and prevention of reactive oxygen species production after hypoglycemia .
Metabolic Regulation in Cancer Cells
DCA is a known activator of pyruvate dehydrogenase (PDH) and inhibitor of pyruvate dehydrogenase kinase (PDK), which are key enzymes in the metabolic pathways of cancer cells . By regulating these enzymes, DCA can potentially alter the metabolic profile of cancer cells and inhibit their growth .
Treatment of Cervical Cancer
In cervical cancer cells, DCA has been shown to increase the sensitivity of these cells to other treatments. For instance, COX2 inhibition by celecoxib makes cervical cancer cells more sensitive to DCA .
General Anticancer Preparation
DCA is an investigational medicinal product that has been employed in experimental and clinical research as a potential anticancer preparation . It has been proposed to cause multiple changes in cancer cells .
Safety And Hazards
Eigenschaften
IUPAC Name |
sodium;2,2-dichloroacetate | |
---|---|---|
Source | PubChem | |
URL | https://pubchem.ncbi.nlm.nih.gov | |
Description | Data deposited in or computed by PubChem | |
InChI |
InChI=1S/C2H2Cl2O2.Na/c3-1(4)2(5)6;/h1H,(H,5,6);/q;+1/p-1/i1+1,2+1; | |
Source | PubChem | |
URL | https://pubchem.ncbi.nlm.nih.gov | |
Description | Data deposited in or computed by PubChem | |
InChI Key |
LUPNKHXLFSSUGS-AWQJXPNKSA-M | |
Source | PubChem | |
URL | https://pubchem.ncbi.nlm.nih.gov | |
Description | Data deposited in or computed by PubChem | |
Canonical SMILES |
C(C(=O)[O-])(Cl)Cl.[Na+] | |
Source | PubChem | |
URL | https://pubchem.ncbi.nlm.nih.gov | |
Description | Data deposited in or computed by PubChem | |
Isomeric SMILES |
[13CH]([13C](=O)[O-])(Cl)Cl.[Na+] | |
Source | PubChem | |
URL | https://pubchem.ncbi.nlm.nih.gov | |
Description | Data deposited in or computed by PubChem | |
Molecular Formula |
C2HCl2NaO2 | |
Source | PubChem | |
URL | https://pubchem.ncbi.nlm.nih.gov | |
Description | Data deposited in or computed by PubChem | |
DSSTOX Substance ID |
DTXSID40745867 | |
Record name | Sodium dichloro(~13~C_2_)acetate | |
Source | EPA DSSTox | |
URL | https://comptox.epa.gov/dashboard/DTXSID40745867 | |
Description | DSSTox provides a high quality public chemistry resource for supporting improved predictive toxicology. | |
Molecular Weight |
152.91 g/mol | |
Source | PubChem | |
URL | https://pubchem.ncbi.nlm.nih.gov | |
Description | Data deposited in or computed by PubChem | |
Product Name |
Sodium dichloroacetate-13C2 | |
CAS RN |
1173022-90-6 | |
Record name | Sodium dichloro(~13~C_2_)acetate | |
Source | EPA DSSTox | |
URL | https://comptox.epa.gov/dashboard/DTXSID40745867 | |
Description | DSSTox provides a high quality public chemistry resource for supporting improved predictive toxicology. | |
Record name | 1173022-90-6 | |
Source | European Chemicals Agency (ECHA) | |
URL | https://echa.europa.eu/information-on-chemicals | |
Description | The European Chemicals Agency (ECHA) is an agency of the European Union which is the driving force among regulatory authorities in implementing the EU's groundbreaking chemicals legislation for the benefit of human health and the environment as well as for innovation and competitiveness. | |
Explanation | Use of the information, documents and data from the ECHA website is subject to the terms and conditions of this Legal Notice, and subject to other binding limitations provided for under applicable law, the information, documents and data made available on the ECHA website may be reproduced, distributed and/or used, totally or in part, for non-commercial purposes provided that ECHA is acknowledged as the source: "Source: European Chemicals Agency, http://echa.europa.eu/". Such acknowledgement must be included in each copy of the material. ECHA permits and encourages organisations and individuals to create links to the ECHA website under the following cumulative conditions: Links can only be made to webpages that provide a link to the Legal Notice page. | |
Haftungsausschluss und Informationen zu In-Vitro-Forschungsprodukten
Bitte beachten Sie, dass alle Artikel und Produktinformationen, die auf BenchChem präsentiert werden, ausschließlich zu Informationszwecken bestimmt sind. Die auf BenchChem zum Kauf angebotenen Produkte sind speziell für In-vitro-Studien konzipiert, die außerhalb lebender Organismen durchgeführt werden. In-vitro-Studien, abgeleitet von dem lateinischen Begriff "in Glas", beinhalten Experimente, die in kontrollierten Laborumgebungen unter Verwendung von Zellen oder Geweben durchgeführt werden. Es ist wichtig zu beachten, dass diese Produkte nicht als Arzneimittel oder Medikamente eingestuft sind und keine Zulassung der FDA für die Vorbeugung, Behandlung oder Heilung von medizinischen Zuständen, Beschwerden oder Krankheiten erhalten haben. Wir müssen betonen, dass jede Form der körperlichen Einführung dieser Produkte in Menschen oder Tiere gesetzlich strikt untersagt ist. Es ist unerlässlich, sich an diese Richtlinien zu halten, um die Einhaltung rechtlicher und ethischer Standards in Forschung und Experiment zu gewährleisten.