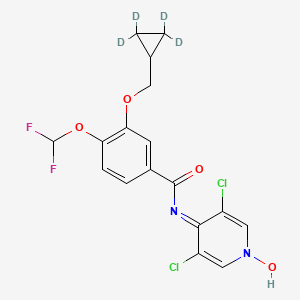
Roflumilast-d4 N-Oxide
Übersicht
Beschreibung
Roflumilast-d4 N-Oxide is a deuterium-labeled derivative of Roflumilast N-Oxide, the primary active metabolite of Roflumilast, a selective phosphodiesterase 4 (PDE4) inhibitor used clinically for chronic obstructive pulmonary disease (COPD). The deuterated form replaces four hydrogen atoms with deuterium, resulting in a molecular formula of C₁₇H₁₀D₄Cl₂F₂N₂O₄ and a molecular weight of 423.23 . It retains the pharmacological activity of its parent compound, specifically targeting PDE4 isoforms (PDE4A1, PDE4A4, PDE4B1, and PDE4B2) with IC₅₀ values of 0.7, 0.9, 0.7, and 0.2 nM, respectively . Its primary application is as a stable isotope-labeled internal standard in pharmacokinetic and metabolic studies, enabling precise quantification of Roflumilast and its metabolites in biological matrices .
Vorbereitungsmethoden
Synthetic Pathways for Roflumilast-d4 N-Oxide
Deuterium Incorporation Strategies
Deuterated compounds like this compound are typically synthesized by introducing stable deuterium isotopes at specific positions during the precursor synthesis. For this compound, deuterium atoms are strategically placed in the parent molecule (roflumilast) before oxidation to the N-oxide form. Common methods include:
-
Isotopic Exchange Reactions : Replacement of hydrogen atoms with deuterium using deuterated solvents (e.g., D₂O) or catalysts under controlled conditions.
-
Deuterated Starting Materials : Use of deuterium-labeled intermediates during the synthesis of roflumilast, ensuring retention of isotopic integrity through subsequent reaction steps .
The chemical structure of this compound (C₁₇H₁₀D₄Cl₂F₂N₂O₄) suggests deuterium incorporation at four non-labile positions, likely in the aromatic or aliphatic regions of the molecule .
Oxidation of Roflumilast-d4 to the N-Oxide
The conversion of roflumilast to its N-oxide metabolite is catalyzed by cytochrome P450 enzymes (CYP3A4 and CYP1A2) in vivo . In vitro, this process is replicated using chemical oxidizing agents:
-
Peracid-Mediated Oxidation : Treatment with meta-chloroperbenzoic acid (mCPBA) in dichloromethane or ethyl acetate at 0–25°C.
-
Hydrogen Peroxide with Catalysts : Use of H₂O₂ in the presence of tungsten-based catalysts under mild acidic conditions .
For the deuterated analog, reaction conditions must be optimized to prevent deuterium loss. Solvent selection (e.g., deuterated chloroform) and low-temperature processing are critical to maintaining isotopic stability .
Crystallization and Polymorph Control
Hydrate Formation and Solvent Systems
The hydrated form of Roflumilast N-Oxide is preferred for pharmaceutical applications due to its enhanced solubility and stability . While data specific to the deuterated form are limited, analogous crystallization methods can be applied:
Parameter | Conditions for Roflumilast N-Oxide Hydrate | Adaptations for Deuterated Form |
---|---|---|
Solvent System | Ethanol-water (1:1 v/v) | Deuterated ethanol (C₂D₅OD)-D₂O |
Temperature | 25–60°C | Controlled to minimize H/D exchange |
Cooling Rate | 0.5°C/min | Similar, with inert atmosphere |
Final Water Content | 2–10 wt% | Monitored via Karl Fischer titration |
Crystalline Form H-1 of Roflumilast N-Oxide, characterized by X-ray diffraction peaks at 5.2, 10.3, and 23.6° 2θ, serves as a reference for optimizing the deuterated analog’s crystal lattice .
Recrystallization Techniques
Recrystallization from a deuterated solvent mixture (e.g., D₂O/methanol-d₄) enhances isotopic purity. Key steps include:
-
Dissolving crude this compound in warm deuterated solvent.
-
Gradual cooling to 4°C to induce nucleation.
-
Vacuum filtration and drying under nitrogen to preserve deuterium content .
Analytical Characterization
Spectroscopic Confirmation
-
Mass Spectrometry (MS) : Electrospray ionization (ESI-MS) confirms the molecular ion peak at m/z 423.23 [M+H]⁺, with isotopic patterning verifying deuterium incorporation .
-
Nuclear Magnetic Resonance (NMR) : ¹H NMR suppression at deuterated positions and ²H NMR signals validate isotopic substitution.
Purity and Stability Assessment
Test | Method | Acceptance Criteria |
---|---|---|
Chemical Purity | HPLC with UV detection (220 nm) | ≥98% area normalization |
Isotopic Enrichment | LC-MS/MS with isotopic standard | ≥99% deuterium at specified sites |
Storage Stability | Accelerated testing (40°C/75% RH) | ≤2% degradation over 6 months |
Stability studies indicate that the hydrated form of Roflumilast N-Oxide retains crystallinity and potency under ambient conditions, a property likely extendable to the deuterated form when stored at -20°C in sealed containers .
Challenges in Deuterated Synthesis
-
Isotopic Dilution : Competing proton sources during synthesis may reduce deuterium content. Strict anhydrous conditions and deuterated reagents mitigate this risk.
-
Regulatory Requirements : Batch-to-batch consistency in isotopic enrichment must meet guidelines for bioanalytical applications (e.g., FDA 2018 Bioanalytical Method Validation).
Analyse Chemischer Reaktionen
Types of Reactions: Roflumilast-d4 N-Oxide undergoes various chemical reactions, including:
Oxidation: The compound can be oxidized to form different metabolites.
Reduction: It can be reduced under specific conditions to yield other derivatives.
Substitution: The deuterium atoms can be replaced with other isotopes or functional groups under certain conditions.
Common Reagents and Conditions:
Oxidation: Common oxidizing agents include hydrogen peroxide and potassium permanganate.
Reduction: Reducing agents such as sodium borohydride and lithium aluminum hydride are often used.
Substitution: Various nucleophiles and electrophiles can be used for substitution reactions.
Major Products Formed: The major products formed from these reactions depend on the specific conditions and reagents used. For example, oxidation may yield different hydroxylated metabolites, while reduction can produce deuterium-labeled alcohols.
Wissenschaftliche Forschungsanwendungen
Roflumilast-d4 N-Oxide is widely used in scientific research, including:
Chemistry: It is used to study the metabolic pathways and degradation products of Roflumilast.
Biology: The compound helps in understanding the biological effects and interactions of Roflumilast in various systems.
Medicine: It is used in pharmacokinetic studies to track the absorption, distribution, metabolism, and excretion of Roflumilast.
Industry: The compound is used in the development of new pharmaceuticals and in quality control processes to ensure the consistency and purity of Roflumilast products.
Wirkmechanismus
Roflumilast-d4 N-Oxide exerts its effects by inhibiting phosphodiesterase 4, an enzyme responsible for breaking down cyclic adenosine monophosphate (cAMP). By inhibiting this enzyme, the compound increases intracellular levels of cAMP, leading to reduced inflammation and other therapeutic effects. The molecular targets include various immune and inflammatory cells, where it modulates the signaling pathways involved in inflammation .
Vergleich Mit ähnlichen Verbindungen
PDE4 Inhibitors
Roflumilast and Roflumilast N-Oxide
- Roflumilast: The parent compound inhibits PDE4 with sub-nanomolar potency (IC₅₀ = 0.7–0.9 nM) and demonstrates anti-inflammatory effects in COPD models. Its oral ED₅₀ for suppressing allergen-induced airway reactions in guinea pigs is 1.5 µmol/kg .
- Roflumilast N-Oxide : The active metabolite exhibits comparable potency (IC₅₀ = 3–40 nM across leukocyte subtypes) and similar oral efficacy (ED₅₀ = 1.0 µmol/kg). Both compounds show 8- to 310-fold greater potency than older PDE4 inhibitors like rolipram and cilomilast .
- Roflumilast-d4 N-Oxide : While pharmacologically identical to Roflumilast N-Oxide, its deuterium labeling enhances metabolic stability, making it invaluable for tracer studies without altering target affinity .
Piclamilast (RP 73401)
- A potent PDE4 inhibitor with IC₅₀ values of 2–13 nM in human leukocytes. However, its oral ED₅₀ in allergen-induced airway models (8.3 µmol/kg ) is 5.5-fold higher than Roflumilast, indicating lower in vivo efficacy .
Rolipram
- A first-generation PDE4 inhibitor with moderate selectivity. Its IC₅₀ varies widely across PDE4 subtypes (e.g., PDE4D IC₅₀ = 1,400 nM ) . In vivo, its ED₅₀ for airway inflammation (32.5 µmol/kg ) is 21.7-fold less potent than Roflumilast .
Cilomilast (Ariflo, SB 207499)
- Exhibits lower potency (IC₅₀ = 40–3,000 nM ) in leukocyte assays and an ED₅₀ of 52.2 µmol/kg in guinea pig models, making it ~35-fold less effective than Roflumilast .
RS-25344 Hydrochloride
- A cAMP-specific PDE4 inhibitor with IC₅₀ = 0.28 nM in human lymphocytes .
Non-PDE4 Inhibitors with Structural or Functional Similarities
S32826 Disodium
- A potent autotaxin inhibitor (IC₅₀ = 8.8 nM ) targeting lipid metabolism rather than PDE4. Its mechanism is distinct from this compound, reflecting divergent therapeutic applications .
Sch59498
- A selective PDE1C inhibitor (IC₅₀ = <10 nM), highlighting the importance of isoform specificity.
Key Pharmacokinetic and Pharmacodynamic Comparisons
Research Findings and Implications
- Superior Potency: this compound and its parent compounds outperform older PDE4 inhibitors in both enzymatic and cellular assays. For example, they inhibit TNF-α production in monocytes with IC₅₀ = 2–21 nM, versus 40–3,000 nM for cilomilast .
- Broad Anti-Inflammatory Activity: Unlike rolipram, which shows variable potency across cell types, this compound exhibits consistent efficacy in neutrophils, eosinophils, monocytes, and T-cells .
- Metabolic Stability : Deuterium labeling in this compound reduces metabolic clearance, extending its utility in tracer studies without compromising target engagement .
Biologische Aktivität
Roflumilast-d4 N-Oxide (RNO) is a derivative of roflumilast, which is primarily known as a phosphodiesterase-4 (PDE4) inhibitor. This compound is particularly significant in the treatment of chronic obstructive pulmonary disease (COPD) and other inflammatory airway diseases. The biological activity of RNO is characterized by its anti-inflammatory properties, pharmacokinetics, and interactions with other therapeutic agents.
Roflumilast and its active metabolite, RNO, exert their effects by inhibiting PDE4, an enzyme that breaks down cyclic adenosine monophosphate (cAMP). Increased levels of cAMP lead to reduced inflammation through various pathways, including the suppression of pro-inflammatory cytokines such as IL-8.
In vitro studies have demonstrated that RNO enhances the anti-inflammatory effects when combined with other medications like formoterol and corticosteroids. For instance, RNO in combination with formoterol significantly increased the repression of IL-8 secretion in airway smooth muscle cells, suggesting a synergistic effect in inflammatory pathways .
2. Pharmacokinetics
The pharmacokinetics of RNO have been studied extensively to understand its absorption, distribution, metabolism, and excretion. Key findings include:
- Steady-State Pharmacokinetics : In patients with varying degrees of liver cirrhosis, the pharmacokinetic profile of RNO showed modest alterations compared to healthy subjects. While roflumilast exposure increased significantly in cirrhotic patients, changes in RNO were less pronounced .
- Comparative Studies : A study comparing the pharmacokinetics between Chinese and Caucasian subjects found that RNO had a higher area under the curve (AUC) and maximum concentration (Cmax) in Chinese participants, indicating potential racial differences in drug metabolism .
The following table summarizes key pharmacokinetic parameters observed in various studies:
Parameter | Healthy Subjects | Child-Pugh A Cirrhosis | Child-Pugh B Cirrhosis |
---|---|---|---|
AUC (Roflumilast) | Baseline | +51% | +92% |
AUC (Roflumilast N-Oxide) | Baseline | +24% | +41% |
Cmax (Roflumilast N-Oxide) | Baseline | +26% | +40% |
3. Anti-inflammatory Activity
The anti-inflammatory effects of RNO are crucial for its therapeutic application in COPD. Studies have shown that both roflumilast and RNO effectively reduce inflammatory cell counts in bronchoalveolar lavage fluid after LPS challenges. Specifically:
- Reduction in Inflammatory Cells : Treatment with roflumilast at 500 mcg daily resulted in significant reductions in neutrophils and eosinophils—key players in airway inflammation—by 38% and 73%, respectively .
- Cytokine Modulation : The ability of RNO to modulate cytokine production highlights its role in managing chronic inflammation associated with respiratory diseases.
4. Case Studies and Clinical Findings
Clinical studies have further elucidated the efficacy of RNO:
- In one open-label study, the combination of RNO with formoterol was shown to enhance anti-inflammatory effects beyond those achieved with corticosteroids alone. This suggests that RNO can be an important adjunct therapy for patients not adequately controlled on corticosteroids .
- Population pharmacokinetic modeling has indicated that factors such as age, sex, race, and smoking status significantly influence the clearance and overall exposure to both roflumilast and RNO, which may inform personalized treatment strategies .
5. Conclusion
This compound demonstrates significant biological activity through its role as a PDE4 inhibitor with pronounced anti-inflammatory effects. Its pharmacokinetic profile varies among populations with different health conditions, suggesting the need for tailored dosing strategies. The combination therapies involving RNO show promise for enhancing treatment outcomes in chronic inflammatory diseases like COPD.
Further research is warranted to explore the full therapeutic potential of RNO and its implications for clinical practice.
Q & A
Basic Research Questions
Q. What validated analytical methods are recommended for quantifying Roflumilast-d4 N-Oxide in biological matrices?
- Methodological Guidance : Liquid chromatography-tandem mass spectrometry (LC-MS/MS) is the gold standard due to its sensitivity and specificity for deuterated compounds. Deuterated internal standards (e.g., Roflumilast-d4) should be used to correct for matrix effects and ionization variability. Ensure calibration curves cover the expected concentration range (e.g., 0.1–100 ng/mL) with precision ≤15% . Detailed protocols must include sample preparation (e.g., protein precipitation, solid-phase extraction), chromatographic conditions (e.g., C18 columns, gradient elution), and mass transition ions (e.g., m/z 407→363 for this compound) .
Q. How is this compound synthesized and characterized to ensure isotopic purity?
- Methodological Guidance : Synthesis involves deuterium incorporation at specific positions (e.g., aromatic rings) via catalytic exchange or deuterated precursor reactions. Characterization requires nuclear magnetic resonance (NMR) to confirm deuterium placement and high-resolution mass spectrometry (HRMS) to verify isotopic purity (>98%). Purity assessment via HPLC-UV (≥95% peak area) and residual solvent analysis (e.g., GC-headspace) are critical for reproducibility . Full synthetic routes and spectral data should be archived in supplementary materials .
Q. What is the role of this compound in studying PDE4 inhibition kinetics?
- Methodological Guidance : As a deuterated metabolite of Roflumilast, it serves as a stable isotope-labeled internal standard in enzymatic assays. Use recombinant PDE4 isoforms (e.g., PDE4B) in vitro with fluorogenic substrates (e.g., cAMP-S-S-FAM) to measure IC50 values. Include controls for non-specific binding and validate inhibition curves with at least three independent replicates. Compare results to non-deuterated Roflumilast N-Oxide to assess isotopic effects on potency .
Advanced Research Questions
Q. How should preclinical studies be designed to assess CYP-mediated drug interactions involving this compound?
- Methodological Guidance : Conduct in vitro microsomal assays (human liver microsomes) to identify CYP isoforms (e.g., CYP1A2, CYP3A4) responsible for metabolism. Use chemical inhibitors (e.g., fluvoxamine for CYP1A2) or recombinant enzymes to confirm pathways. In vivo, administer this compound with CYP inhibitors (e.g., enoxacin) in rodent models and measure plasma exposure (AUC, Cmax) via LC-MS/MS. Follow NIH guidelines for preclinical reporting, including sample size justification and statistical power analysis .
Q. How can researchers resolve contradictions in reported PDE4 inhibition data for this compound across studies?
- Methodological Guidance : Discrepancies in IC50 values may arise from assay conditions (e.g., substrate concentration, enzyme sources). Perform meta-analyses to identify confounding variables and conduct side-by-side assays under standardized conditions (e.g., uniform buffer pH, temperature). Use ANOVA to compare datasets and apply the FINER criteria (Feasible, Novel, Ethical, Relevant) to refine hypotheses . Publish raw data in repositories to enable cross-validation .
Q. What strategies ensure deuterium stability in this compound during long-term storage and in vivo experiments?
- Methodological Guidance : Store lyophilized powder at -80°C under argon to prevent deuterium exchange with ambient moisture. For in vivo studies, validate stability by comparing pre- and post-administration plasma samples using HRMS. Monitor for deuterium loss (>2% variability) and adjust formulation (e.g., lipid-based carriers) if necessary . Report storage conditions and stability data in experimental sections .
Q. How can FAIR data principles be applied to studies involving this compound?
- Methodological Guidance :
- Findable : Assign persistent identifiers (e.g., DOI) to datasets and metadata in repositories like Chemotion or Zenodo.
- Accessible : Use open-access formats (e.g., .csv for raw LC-MS data) and provide README files with experimental details.
- Interoperable : Adopt standardized ontologies (e.g., ChEBI for chemical identifiers) and align with ISA-Tab metadata frameworks.
- Reusable : Document synthesis protocols, instrument settings, and statistical codes in supplementary materials .
Eigenschaften
IUPAC Name |
N-(3,5-dichloro-1-hydroxypyridin-4-ylidene)-4-(difluoromethoxy)-3-[(2,2,3,3-tetradeuteriocyclopropyl)methoxy]benzamide | |
---|---|---|
Source | PubChem | |
URL | https://pubchem.ncbi.nlm.nih.gov | |
Description | Data deposited in or computed by PubChem | |
InChI |
InChI=1S/C17H14Cl2F2N2O4/c18-11-6-23(25)7-12(19)15(11)22-16(24)10-3-4-13(27-17(20)21)14(5-10)26-8-9-1-2-9/h3-7,9,17,25H,1-2,8H2/i1D2,2D2 | |
Source | PubChem | |
URL | https://pubchem.ncbi.nlm.nih.gov | |
Description | Data deposited in or computed by PubChem | |
InChI Key |
KHXXMSARUQULRI-LNLMKGTHSA-N | |
Source | PubChem | |
URL | https://pubchem.ncbi.nlm.nih.gov | |
Description | Data deposited in or computed by PubChem | |
Canonical SMILES |
C1CC1COC2=C(C=CC(=C2)C(=O)N=C3C(=CN(C=C3Cl)O)Cl)OC(F)F | |
Source | PubChem | |
URL | https://pubchem.ncbi.nlm.nih.gov | |
Description | Data deposited in or computed by PubChem | |
Isomeric SMILES |
[2H]C1(C(C1([2H])[2H])COC2=C(C=CC(=C2)C(=O)N=C3C(=CN(C=C3Cl)O)Cl)OC(F)F)[2H] | |
Source | PubChem | |
URL | https://pubchem.ncbi.nlm.nih.gov | |
Description | Data deposited in or computed by PubChem | |
Molecular Formula |
C17H14Cl2F2N2O4 | |
Source | PubChem | |
URL | https://pubchem.ncbi.nlm.nih.gov | |
Description | Data deposited in or computed by PubChem | |
Molecular Weight |
423.2 g/mol | |
Source | PubChem | |
URL | https://pubchem.ncbi.nlm.nih.gov | |
Description | Data deposited in or computed by PubChem | |
Haftungsausschluss und Informationen zu In-Vitro-Forschungsprodukten
Bitte beachten Sie, dass alle Artikel und Produktinformationen, die auf BenchChem präsentiert werden, ausschließlich zu Informationszwecken bestimmt sind. Die auf BenchChem zum Kauf angebotenen Produkte sind speziell für In-vitro-Studien konzipiert, die außerhalb lebender Organismen durchgeführt werden. In-vitro-Studien, abgeleitet von dem lateinischen Begriff "in Glas", beinhalten Experimente, die in kontrollierten Laborumgebungen unter Verwendung von Zellen oder Geweben durchgeführt werden. Es ist wichtig zu beachten, dass diese Produkte nicht als Arzneimittel oder Medikamente eingestuft sind und keine Zulassung der FDA für die Vorbeugung, Behandlung oder Heilung von medizinischen Zuständen, Beschwerden oder Krankheiten erhalten haben. Wir müssen betonen, dass jede Form der körperlichen Einführung dieser Produkte in Menschen oder Tiere gesetzlich strikt untersagt ist. Es ist unerlässlich, sich an diese Richtlinien zu halten, um die Einhaltung rechtlicher und ethischer Standards in Forschung und Experiment zu gewährleisten.