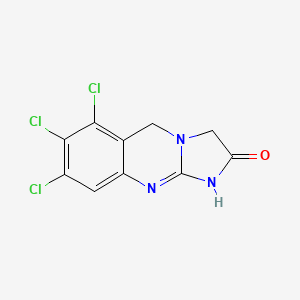
Anagrelide trichloro derivative
Übersicht
Beschreibung
Anagrelide trichloro derivative is a chemical compound with the CAS number 1092352-99-2 . Its chemical name is 6,7,8-Trichloro-3,5-dihydroimidazo[2,1-b]quinazolin-2(1H)-one . It is also known by the synonym 8-Chloro Anagrelide .
Molecular Structure Analysis
The molecular formula of Anagrelide trichloro derivative is C10H6Cl3N3O . The molecular weight is 290.5 . The structure can be represented by the SMILES notation: O=C1NC2=NC3=C(C(Cl)=C(Cl)C(Cl)=C3)CN2C1 .Wissenschaftliche Forschungsanwendungen
Essential Thrombocythemia Treatment
8-Chloro Anagrelide is primarily used in the treatment of essential thrombocythemia (ET), a condition characterized by the overproduction of platelets. It acts as a cytoreductive therapy, effectively reducing platelet counts and mitigating the risk of thrombohemorrhagic events . In Japan, it has been studied as a second-line treatment for high-risk patients intolerant or refractory to first-line therapies .
Platelet Count Reduction in Myeloproliferative Neoplasms
The compound is also indicated for use in myeloproliferative neoplasms, where it helps lower dangerously elevated platelet levels. Its mechanism of action involves the inhibition of phosphodiesterase activity, particularly phosphodiesterase-III, which leads to decreased platelet production in the bone marrow .
Chronic Myeloid Leukemia
Anagrelide has been utilized in the treatment of chronic myeloid leukemia (CML). While not the primary treatment, it serves as an adjunct therapy to control platelet counts in patients suffering from CML, providing symptomatic relief and preventing complications associated with high platelet numbers .
Pharmacological Agent in Platelet Level Management
Chemically identified as a potent pharmacological agent, 8-Chloro Anagrelide is recognized for its capacity to significantly reduce blood platelet levels. This property is crucial for managing conditions like essential thrombocytosis, where platelet levels need to be controlled to prevent thrombotic events .
Drug Metabolism and Pharmacokinetics Studies
The compound’s role extends to drug metabolism and pharmacokinetics studies. Its unique chemical structure and properties make it a subject of interest in understanding how drugs are metabolized and processed within the body, which is essential for the development of safe and effective medications .
Wirkmechanismus
- By inhibiting PDE-III, anagrelide increases intracellular cAMP levels, leading to downstream effects on platelet formation .
- The exact mechanism remains unclear, but it involves inhibition of phosphodiesterase A2 (PLA2) and suppression of arachidonic acid release .
- Food intake impacts AER pharmacokinetics, affecting Cmax, AUC, Tmax, T1/2, plateau, and mean residence time .
- Anagrelide does not alter platelet survival but specifically blocks megakaryocyte differentiation and proliferation .
Target of Action
Mode of Action
Biochemical Pathways
Pharmacokinetics
Result of Action
Action Environment
Zukünftige Richtungen
While specific future directions for Anagrelide trichloro derivative are not mentioned, Anagrelide is being studied for its efficacy and safety in cytoreduction therapy-naïve essential thrombocythemia (ET) patients . This suggests potential future research directions for Anagrelide trichloro derivative as well.
Eigenschaften
IUPAC Name |
6,7,8-trichloro-3,5-dihydro-1H-imidazo[2,1-b]quinazolin-2-one | |
---|---|---|
Source | PubChem | |
URL | https://pubchem.ncbi.nlm.nih.gov | |
Description | Data deposited in or computed by PubChem | |
InChI |
InChI=1S/C10H6Cl3N3O/c11-5-1-6-4(8(12)9(5)13)2-16-3-7(17)15-10(16)14-6/h1H,2-3H2,(H,14,15,17) | |
Source | PubChem | |
URL | https://pubchem.ncbi.nlm.nih.gov | |
Description | Data deposited in or computed by PubChem | |
InChI Key |
QCMDDIIKBFVZSY-UHFFFAOYSA-N | |
Source | PubChem | |
URL | https://pubchem.ncbi.nlm.nih.gov | |
Description | Data deposited in or computed by PubChem | |
Canonical SMILES |
C1C2=C(C(=C(C=C2N=C3N1CC(=O)N3)Cl)Cl)Cl | |
Source | PubChem | |
URL | https://pubchem.ncbi.nlm.nih.gov | |
Description | Data deposited in or computed by PubChem | |
Molecular Formula |
C10H6Cl3N3O | |
Source | PubChem | |
URL | https://pubchem.ncbi.nlm.nih.gov | |
Description | Data deposited in or computed by PubChem | |
DSSTOX Substance ID |
DTXSID20148852 | |
Record name | Anagrelide trichloro derivative | |
Source | EPA DSSTox | |
URL | https://comptox.epa.gov/dashboard/DTXSID20148852 | |
Description | DSSTox provides a high quality public chemistry resource for supporting improved predictive toxicology. | |
Molecular Weight |
290.5 g/mol | |
Source | PubChem | |
URL | https://pubchem.ncbi.nlm.nih.gov | |
Description | Data deposited in or computed by PubChem | |
CAS RN |
1092352-99-2 | |
Record name | 6,7,8-Trichloro-1,5-dihydroimidazo[2,1-b]quinazolin-2(3H)-one | |
Source | CAS Common Chemistry | |
URL | https://commonchemistry.cas.org/detail?cas_rn=1092352-99-2 | |
Description | CAS Common Chemistry is an open community resource for accessing chemical information. Nearly 500,000 chemical substances from CAS REGISTRY cover areas of community interest, including common and frequently regulated chemicals, and those relevant to high school and undergraduate chemistry classes. This chemical information, curated by our expert scientists, is provided in alignment with our mission as a division of the American Chemical Society. | |
Explanation | The data from CAS Common Chemistry is provided under a CC-BY-NC 4.0 license, unless otherwise stated. | |
Record name | Anagrelide trichloro derivative | |
Source | ChemIDplus | |
URL | https://pubchem.ncbi.nlm.nih.gov/substance/?source=chemidplus&sourceid=1092352992 | |
Description | ChemIDplus is a free, web search system that provides access to the structure and nomenclature authority files used for the identification of chemical substances cited in National Library of Medicine (NLM) databases, including the TOXNET system. | |
Record name | Anagrelide trichloro derivative | |
Source | EPA DSSTox | |
URL | https://comptox.epa.gov/dashboard/DTXSID20148852 | |
Description | DSSTox provides a high quality public chemistry resource for supporting improved predictive toxicology. | |
Record name | 8-CHLOROANAGRELIDE | |
Source | FDA Global Substance Registration System (GSRS) | |
URL | https://gsrs.ncats.nih.gov/ginas/app/beta/substances/4AZ855T0I6 | |
Description | The FDA Global Substance Registration System (GSRS) enables the efficient and accurate exchange of information on what substances are in regulated products. Instead of relying on names, which vary across regulatory domains, countries, and regions, the GSRS knowledge base makes it possible for substances to be defined by standardized, scientific descriptions. | |
Explanation | Unless otherwise noted, the contents of the FDA website (www.fda.gov), both text and graphics, are not copyrighted. They are in the public domain and may be republished, reprinted and otherwise used freely by anyone without the need to obtain permission from FDA. Credit to the U.S. Food and Drug Administration as the source is appreciated but not required. | |
Haftungsausschluss und Informationen zu In-Vitro-Forschungsprodukten
Bitte beachten Sie, dass alle Artikel und Produktinformationen, die auf BenchChem präsentiert werden, ausschließlich zu Informationszwecken bestimmt sind. Die auf BenchChem zum Kauf angebotenen Produkte sind speziell für In-vitro-Studien konzipiert, die außerhalb lebender Organismen durchgeführt werden. In-vitro-Studien, abgeleitet von dem lateinischen Begriff "in Glas", beinhalten Experimente, die in kontrollierten Laborumgebungen unter Verwendung von Zellen oder Geweben durchgeführt werden. Es ist wichtig zu beachten, dass diese Produkte nicht als Arzneimittel oder Medikamente eingestuft sind und keine Zulassung der FDA für die Vorbeugung, Behandlung oder Heilung von medizinischen Zuständen, Beschwerden oder Krankheiten erhalten haben. Wir müssen betonen, dass jede Form der körperlichen Einführung dieser Produkte in Menschen oder Tiere gesetzlich strikt untersagt ist. Es ist unerlässlich, sich an diese Richtlinien zu halten, um die Einhaltung rechtlicher und ethischer Standards in Forschung und Experiment zu gewährleisten.