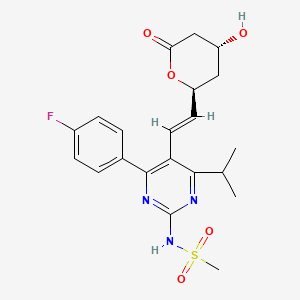
N-Desmethyl Rosuvastatin Lacton
- Klicken Sie auf QUICK INQUIRY, um ein Angebot von unserem Expertenteam zu erhalten.
- Mit qualitativ hochwertigen Produkten zu einem WETTBEWERBSFÄHIGEN Preis können Sie sich mehr auf Ihre Forschung konzentrieren.
Übersicht
Beschreibung
N-Desmethyl Rosuvastatin Lactone is a metabolite of Rosuvastatin, a widely used statin for lowering cholesterol levels. This compound is formed through the metabolic processes involving Rosuvastatin and plays a role in the pharmacokinetics and pharmacodynamics of the parent drug .
Wissenschaftliche Forschungsanwendungen
N-Desmethyl Rosuvastatin Lactone has several scientific research applications:
Wirkmechanismus
Target of Action
N-Desmethyl Rosuvastatin Lactone, like its parent compound Rosuvastatin, primarily targets the enzyme 3-hydroxy-3-methylglutaryl coenzyme A (HMG-CoA) reductase . This enzyme plays a crucial role in the endogenous production of cholesterol in the liver .
Mode of Action
N-Desmethyl Rosuvastatin Lactone acts as a competitive inhibitor of HMG-CoA reductase . By inhibiting this enzyme, it reduces the conversion of HMG-CoA to mevalonate, an early and rate-limiting step in cholesterol biosynthesis . This action primarily occurs in the liver .
Biochemical Pathways
The inhibition of HMG-CoA reductase leads to a decrease in the production of several compounds involved in lipid metabolism and transport, including cholesterol, low-density lipoprotein (LDL), and very low-density lipoprotein (VLDL) . This results in a compensatory increase in the expression of LDL receptors on hepatocyte membranes and a stimulation of LDL catabolism .
Pharmacokinetics
Rosuvastatin, and by extension N-Desmethyl Rosuvastatin Lactone, exhibits variable pharmacokinetics across studies . It is given once daily, achieving maximum plasma concentration at a median of 5 hours under fasting conditions following single and multiple doses . The systemic exposure of rosuvastatin is characterized by a large coefficient of variation . There is a small accumulation with repeated dosing . The overall mean total clearance of the drug in Caucasian subjects was found to be 1.7-fold higher than that in healthy Chinese subjects .
Result of Action
The primary result of the action of N-Desmethyl Rosuvastatin Lactone is a reduction in the levels of LDL cholesterol, total cholesterol, and triglycerides . This leads to a decreased risk of cardiovascular disease, including myocardial infarction and stroke .
Action Environment
The action, efficacy, and stability of N-Desmethyl Rosuvastatin Lactone can be influenced by various environmental factors. For instance, the pharmacokinetics of rosuvastatin shows considerable variation between races . Additionally, certain drug interactions can significantly affect the action of rosuvastatin . For example, the interaction of rosuvastatin with darunavir/ritonavir was considered statistically and clinically relevant .
Vorbereitungsmethoden
Synthetic Routes and Reaction Conditions
The synthesis of N-Desmethyl Rosuvastatin Lactone involves the demethylation of Rosuvastatin followed by lactonization. The demethylation process is typically catalyzed by cytochrome P450 enzymes, particularly CYP2C9 . The lactonization step involves the formation of a lactone ring, which can be facilitated by acidic conditions .
Industrial Production Methods
Industrial production of N-Desmethyl Rosuvastatin Lactone follows similar synthetic routes but on a larger scale. The process involves optimizing reaction conditions to maximize yield and purity. Techniques such as liquid chromatography-tandem mass spectrometry (LC-MS/MS) are used for the quantification and quality control of the compound .
Analyse Chemischer Reaktionen
Types of Reactions
N-Desmethyl Rosuvastatin Lactone undergoes various chemical reactions, including:
Oxidation: This reaction can be facilitated by oxidizing agents, leading to the formation of oxidized derivatives.
Reduction: Reduction reactions can convert the lactone back to its corresponding hydroxy acid form.
Substitution: Nucleophilic substitution reactions can occur at specific sites on the molecule.
Common Reagents and Conditions
Oxidation: Common oxidizing agents include hydrogen peroxide and potassium permanganate.
Reduction: Reducing agents such as sodium borohydride are used.
Substitution: Nucleophiles like amines and thiols can be used under basic conditions.
Major Products Formed
The major products formed from these reactions include various oxidized and reduced derivatives, as well as substituted analogs of N-Desmethyl Rosuvastatin Lactone .
Vergleich Mit ähnlichen Verbindungen
Similar Compounds
Rosuvastatin: The parent compound, which is more potent in inhibiting HMG-CoA reductase.
Rosuvastatin-5S-Lactone: Another metabolite of Rosuvastatin, which lacks HMG-CoA reductase inhibitory activity.
N-Desmethyl Rosuvastatin: A direct metabolite of Rosuvastatin, formed by demethylation.
Uniqueness
N-Desmethyl Rosuvastatin Lactone is unique due to its specific metabolic pathway and the formation of a lactone ring, which distinguishes it from other metabolites of Rosuvastatin. Its presence and concentration in the plasma can provide insights into the metabolic fate of Rosuvastatin and its overall pharmacokinetic profile .
Biologische Aktivität
N-Desmethyl Rosuvastatin Lactone (DM-RSL) is a significant metabolite of Rosuvastatin, a widely prescribed statin for managing hypercholesterolemia and cardiovascular diseases. Understanding the biological activity of DM-RSL is crucial for grasping the pharmacological effects of Rosuvastatin and its derivatives.
N-Desmethyl Rosuvastatin Lactone is characterized by its lactone structure, which plays a vital role in its biological functions. The compound has a molecular weight of approximately 455.5 g/mol and is produced through the metabolic pathways involving cytochrome P450 enzymes, particularly CYP2C9, CYP2C19, and CYP3A4 .
Biological Activities
1. Lipid Metabolism:
DM-RSL retains lipid-lowering properties similar to those of Rosuvastatin. It has been shown to reduce levels of low-density lipoprotein (LDL) cholesterol while increasing high-density lipoprotein (HDL) cholesterol. This effect is primarily mediated through the inhibition of HMG-CoA reductase, the enzyme critical for cholesterol biosynthesis .
2. Anti-inflammatory Effects:
Research indicates that DM-RSL exhibits anti-inflammatory activities by reducing the secretion of pro-inflammatory cytokines such as interleukin-6 (IL-6) and interleukin-8 (IL-8) in various cell culture studies . This property may contribute to its cardiovascular protective mechanisms, as inflammation plays a significant role in atherosclerosis.
3. Drug-Drug Interactions:
DM-RSL's interaction with cytochrome P450 enzymes suggests potential drug-drug interactions that could influence the metabolism of concurrent medications. Understanding these interactions is essential for optimizing therapeutic regimens involving statins .
Case Studies and Clinical Trials
Several studies have explored the pharmacokinetics and biological effects of DM-RSL:
- Pharmacokinetic Analysis: A study assessing the concentrations of Rosuvastatin and its metabolites (including DM-RSL) demonstrated that circulating levels of DM-RSL were significantly lower than those of Rosuvastatin after administration, indicating that while it is an active metabolite, it does not accumulate to the same extent .
- Clinical Application: A clinical trial utilized liquid chromatography-tandem mass spectrometry (LC-MS/MS) to quantify DM-RSL in human plasma, confirming its presence and providing insights into its metabolic profile post-Rosuvastatin administration .
Comparative Analysis with Other Statins
The following table compares DM-RSL with other statins and their metabolites regarding their structural characteristics and biological activities:
Compound Name | Structure Type | Unique Features |
---|---|---|
Rosuvastatin | Hydroxy acid | Potent LDL-cholesterol lowering effects |
Simvastatin | Lactone | Strong lipid-lowering properties; different metabolic profile |
Atorvastatin | Hydroxy acid | Similar mechanism; distinct side effects |
N-Desmethyl Atorvastatin | Metabolite | Affects bioavailability |
Lovastatin | Lactone | First statin discovered; less potent than newer agents |
N-Desmethyl Rosuvastatin | Metabolite | Active metabolite contributing to lipid-lowering effects |
Eigenschaften
IUPAC Name |
N-[4-(4-fluorophenyl)-5-[(E)-2-[(2S,4R)-4-hydroxy-6-oxooxan-2-yl]ethenyl]-6-propan-2-ylpyrimidin-2-yl]methanesulfonamide |
Source
|
---|---|---|
Source | PubChem | |
URL | https://pubchem.ncbi.nlm.nih.gov | |
Description | Data deposited in or computed by PubChem | |
InChI |
InChI=1S/C21H24FN3O5S/c1-12(2)19-17(9-8-16-10-15(26)11-18(27)30-16)20(13-4-6-14(22)7-5-13)24-21(23-19)25-31(3,28)29/h4-9,12,15-16,26H,10-11H2,1-3H3,(H,23,24,25)/b9-8+/t15-,16-/m1/s1 |
Source
|
Source | PubChem | |
URL | https://pubchem.ncbi.nlm.nih.gov | |
Description | Data deposited in or computed by PubChem | |
InChI Key |
WSCHZXKSCWERQN-GUFYHEMZSA-N |
Source
|
Source | PubChem | |
URL | https://pubchem.ncbi.nlm.nih.gov | |
Description | Data deposited in or computed by PubChem | |
Canonical SMILES |
CC(C)C1=NC(=NC(=C1C=CC2CC(CC(=O)O2)O)C3=CC=C(C=C3)F)NS(=O)(=O)C |
Source
|
Source | PubChem | |
URL | https://pubchem.ncbi.nlm.nih.gov | |
Description | Data deposited in or computed by PubChem | |
Isomeric SMILES |
CC(C)C1=NC(=NC(=C1/C=C/[C@@H]2C[C@H](CC(=O)O2)O)C3=CC=C(C=C3)F)NS(=O)(=O)C |
Source
|
Source | PubChem | |
URL | https://pubchem.ncbi.nlm.nih.gov | |
Description | Data deposited in or computed by PubChem | |
Molecular Formula |
C21H24FN3O5S |
Source
|
Source | PubChem | |
URL | https://pubchem.ncbi.nlm.nih.gov | |
Description | Data deposited in or computed by PubChem | |
Molecular Weight |
449.5 g/mol |
Source
|
Source | PubChem | |
URL | https://pubchem.ncbi.nlm.nih.gov | |
Description | Data deposited in or computed by PubChem | |
Haftungsausschluss und Informationen zu In-Vitro-Forschungsprodukten
Bitte beachten Sie, dass alle Artikel und Produktinformationen, die auf BenchChem präsentiert werden, ausschließlich zu Informationszwecken bestimmt sind. Die auf BenchChem zum Kauf angebotenen Produkte sind speziell für In-vitro-Studien konzipiert, die außerhalb lebender Organismen durchgeführt werden. In-vitro-Studien, abgeleitet von dem lateinischen Begriff "in Glas", beinhalten Experimente, die in kontrollierten Laborumgebungen unter Verwendung von Zellen oder Geweben durchgeführt werden. Es ist wichtig zu beachten, dass diese Produkte nicht als Arzneimittel oder Medikamente eingestuft sind und keine Zulassung der FDA für die Vorbeugung, Behandlung oder Heilung von medizinischen Zuständen, Beschwerden oder Krankheiten erhalten haben. Wir müssen betonen, dass jede Form der körperlichen Einführung dieser Produkte in Menschen oder Tiere gesetzlich strikt untersagt ist. Es ist unerlässlich, sich an diese Richtlinien zu halten, um die Einhaltung rechtlicher und ethischer Standards in Forschung und Experiment zu gewährleisten.