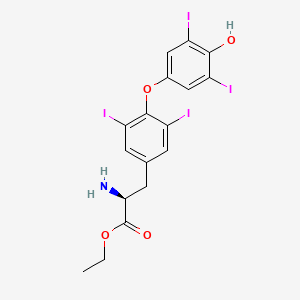
Thyroxine Ethyl Ester
Übersicht
Beschreibung
Thyroxine Ethyl Ester is a synthetic derivative of thyroxine, a hormone produced by the thyroid gland. Thyroxine, also known as tetraiodothyronine, plays a crucial role in regulating metabolism, growth, and development in humans. This compound is often used in scientific research due to its stability and ease of handling compared to its parent compound.
Wissenschaftliche Forschungsanwendungen
Thyroxine Ethyl Ester is widely used in scientific research due to its stability and ease of handling. Some of its applications include:
Chemistry: It is used as a reference standard in analytical chemistry for the quantification of thyroxine and its derivatives.
Biology: It serves as a tool to study thyroid hormone metabolism and its effects on cellular processes.
Medicine: Research on this compound helps in understanding thyroid hormone replacement therapies and their pharmacokinetics.
Industry: It is used in the development of diagnostic assays for thyroid function testing.
Wirkmechanismus
- TRs exist in two isoforms: TRα and TRβ. TRα is mainly found in cardiac tissue, skeletal muscle, and the brain, while TRβ is prevalent in the liver, kidney, and thyroid gland .
- T3 binds to TRs in the nucleus, leading to gene transcription. This process affects metabolism, growth, and development .
- TEE influences several pathways:
Target of Action
Mode of Action
Biochemical Pathways
Pharmacokinetics
Result of Action
Safety and Hazards
Zukünftige Richtungen
Biochemische Analyse
Biochemical Properties
Thyroxine Ethyl Ester plays a role in various biochemical reactions . It interacts with enzymes, proteins, and other biomolecules, influencing their function and activity . For instance, it is known to interact with deiodinase enzymes, which are responsible for the conversion of thyroxine (T4) into the more active form, triiodothyronine (T3)
Cellular Effects
This compound has significant effects on various types of cells and cellular processes . It influences cell function by impacting cell signaling pathways, gene expression, and cellular metabolism . For example, increased levels of thyroid hormones, such as this compound, can improve serum lipid profiles and reduce body fat . These positive effects are counterbalanced by potential adverse effects on the heart, muscle, and bone .
Molecular Mechanism
The molecular mechanism of action of this compound involves binding interactions with biomolecules, enzyme inhibition or activation, and changes in gene expression . This compound exerts its effects at the molecular level, primarily through its conversion to T3, the active form of thyroid hormone . This process involves the removal of an iodine atom, a reaction catalyzed by deiodinase enzymes .
Temporal Effects in Laboratory Settings
In laboratory settings, the effects of this compound change over time . Studies have shown that the product’s stability, degradation, and long-term effects on cellular function can be observed in both in vitro and in vivo studies . For instance, temporal toxic exposure to thyroxine has been shown to have benign clinical courses due to low FT3/FT4 ratios .
Dosage Effects in Animal Models
The effects of this compound vary with different dosages in animal models . Studies have shown that thyroid hormones can improve metabolic profiles at certain dosages, but may also have toxic or adverse effects at high doses . Understanding these dosage effects is crucial for determining safe and effective therapeutic applications.
Metabolic Pathways
This compound is involved in several metabolic pathways . It interacts with various enzymes and cofactors, and can influence metabolic flux or metabolite levels . For example, thyroid hormones are known to stimulate calorigenesis/thermogenesis by affecting cellular respiration .
Transport and Distribution
This compound is transported and distributed within cells and tissues . It interacts with transporters such as the Organic Acid Transporting Polypeptide 2B1 (OATP2B1), which facilitates the cellular uptake of thyroid hormones . The distribution of this compound can also influence its localization or accumulation within cells .
Subcellular Localization
The subcellular localization of this compound can affect its activity or function . Studies have shown that thyroxine-5′-deiodinase, an enzyme involved in the conversion of thyroxine to triiodothyronine, is localized solely in the microsomes of rat liver . This suggests that this compound may also be localized in specific compartments or organelles within the cell, influencing its activity and function .
Vorbereitungsmethoden
Synthetic Routes and Reaction Conditions: Thyroxine Ethyl Ester can be synthesized through the esterification of thyroxine. The process involves dissolving thyroxine in ethanol and adding hydrochloric acid to catalyze the reaction. The mixture is then heated, and the solvent is slowly evaporated to yield this compound .
Industrial Production Methods: Industrial production of this compound follows a similar process but on a larger scale. The reaction conditions are optimized to ensure high yield and purity. The use of continuous reactors and advanced purification techniques helps in achieving the desired product quality .
Analyse Chemischer Reaktionen
Types of Reactions: Thyroxine Ethyl Ester undergoes various chemical reactions, including:
Oxidation: It can be oxidized to form different iodinated derivatives.
Reduction: Reduction reactions can lead to the formation of deiodinated products.
Substitution: Halogen substitution reactions can occur, leading to the formation of various analogs.
Common Reagents and Conditions:
Oxidation: Common oxidizing agents include hydrogen peroxide and iodine.
Reduction: Reducing agents such as sodium borohydride are used.
Substitution: Halogenation reactions often use reagents like iodine monochloride.
Major Products: The major products formed from these reactions include various iodinated and deiodinated derivatives of this compound .
Vergleich Mit ähnlichen Verbindungen
Levothyroxine: A synthetic form of thyroxine used in thyroid hormone replacement therapy.
Liothyronine: A synthetic form of triiodothyronine, another thyroid hormone.
Diiodothyronine: A metabolite of thyroxine with distinct biological activities.
Comparison: Thyroxine Ethyl Ester is unique due to its esterified form, which provides greater stability and ease of handling compared to thyroxine. Unlike Levothyroxine and Liothyronine, which are commonly used in clinical settings, this compound is primarily used in research applications .
Eigenschaften
IUPAC Name |
ethyl (2S)-2-amino-3-[4-(4-hydroxy-3,5-diiodophenoxy)-3,5-diiodophenyl]propanoate | |
---|---|---|
Source | PubChem | |
URL | https://pubchem.ncbi.nlm.nih.gov | |
Description | Data deposited in or computed by PubChem | |
InChI |
InChI=1S/C17H15I4NO4/c1-2-25-17(24)14(22)5-8-3-12(20)16(13(21)4-8)26-9-6-10(18)15(23)11(19)7-9/h3-4,6-7,14,23H,2,5,22H2,1H3/t14-/m0/s1 | |
Source | PubChem | |
URL | https://pubchem.ncbi.nlm.nih.gov | |
Description | Data deposited in or computed by PubChem | |
InChI Key |
LSSGNEFHGVQOPA-AWEZNQCLSA-N | |
Source | PubChem | |
URL | https://pubchem.ncbi.nlm.nih.gov | |
Description | Data deposited in or computed by PubChem | |
Canonical SMILES |
CCOC(=O)C(CC1=CC(=C(C(=C1)I)OC2=CC(=C(C(=C2)I)O)I)I)N | |
Source | PubChem | |
URL | https://pubchem.ncbi.nlm.nih.gov | |
Description | Data deposited in or computed by PubChem | |
Isomeric SMILES |
CCOC(=O)[C@H](CC1=CC(=C(C(=C1)I)OC2=CC(=C(C(=C2)I)O)I)I)N | |
Source | PubChem | |
URL | https://pubchem.ncbi.nlm.nih.gov | |
Description | Data deposited in or computed by PubChem | |
Molecular Formula |
C17H15I4NO4 | |
Source | PubChem | |
URL | https://pubchem.ncbi.nlm.nih.gov | |
Description | Data deposited in or computed by PubChem | |
DSSTOX Substance ID |
DTXSID20708672 | |
Record name | Ethyl O-(4-hydroxy-3,5-diiodophenyl)-3,5-diiodo-L-tyrosinate | |
Source | EPA DSSTox | |
URL | https://comptox.epa.gov/dashboard/DTXSID20708672 | |
Description | DSSTox provides a high quality public chemistry resource for supporting improved predictive toxicology. | |
Molecular Weight |
804.92 g/mol | |
Source | PubChem | |
URL | https://pubchem.ncbi.nlm.nih.gov | |
Description | Data deposited in or computed by PubChem | |
CAS No. |
76353-71-4 | |
Record name | Ethyl O-(4-hydroxy-3,5-diiodophenyl)-3,5-diiodo-L-tyrosinate | |
Source | EPA DSSTox | |
URL | https://comptox.epa.gov/dashboard/DTXSID20708672 | |
Description | DSSTox provides a high quality public chemistry resource for supporting improved predictive toxicology. | |
Q1: What is the primary effect of D,L-α-Methyl Thyroxine Ethyl Ester (CG-635) investigated in the provided research?
A1: The primary focus of the research is exploring the lipid-lowering effect of D,L-α-Methyl this compound (CG-635) [, ]. This suggests the compound might play a role in managing lipid levels, potentially offering therapeutic benefits for conditions like hyperlipidemia.
Q2: Besides its lipid-lowering effect, what other aspects of D,L-α-Methyl this compound (CG-635) are studied in the research?
A2: The research also investigates the influence of D,L-α-Methyl this compound (CG-635) on basal metabolic rate, heart, and thyroid gland [, ]. This implies that the compound's effects are not limited to lipid metabolism and could have broader physiological implications.
Haftungsausschluss und Informationen zu In-Vitro-Forschungsprodukten
Bitte beachten Sie, dass alle Artikel und Produktinformationen, die auf BenchChem präsentiert werden, ausschließlich zu Informationszwecken bestimmt sind. Die auf BenchChem zum Kauf angebotenen Produkte sind speziell für In-vitro-Studien konzipiert, die außerhalb lebender Organismen durchgeführt werden. In-vitro-Studien, abgeleitet von dem lateinischen Begriff "in Glas", beinhalten Experimente, die in kontrollierten Laborumgebungen unter Verwendung von Zellen oder Geweben durchgeführt werden. Es ist wichtig zu beachten, dass diese Produkte nicht als Arzneimittel oder Medikamente eingestuft sind und keine Zulassung der FDA für die Vorbeugung, Behandlung oder Heilung von medizinischen Zuständen, Beschwerden oder Krankheiten erhalten haben. Wir müssen betonen, dass jede Form der körperlichen Einführung dieser Produkte in Menschen oder Tiere gesetzlich strikt untersagt ist. Es ist unerlässlich, sich an diese Richtlinien zu halten, um die Einhaltung rechtlicher und ethischer Standards in Forschung und Experiment zu gewährleisten.