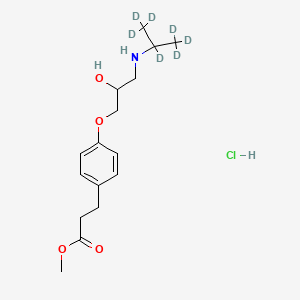
Esmolol-d7 Hydrochloride
Übersicht
Beschreibung
Esmolol-d7 Hydrochloride is a cardioselective beta-adrenergic blocker . It is used for the short-term control of ventricular rate and heart rate in various types of tachycardia, including perioperative tachycardia and hypertension . It is also a class II antiarrhythmic drug used to decrease the force and rate of heart contractions during surgery .
Synthesis Analysis
In the synthesis of Esmolol hydrochloride, four prominent process impurities were formed . These impurities were detected in gradient HPLC method . They are synthesized in different synthesis methods and characterized as Esmolol free acid, Esmolol isopropyl amide analog, N-Ethyl Esmolol, and Esmolol dimer by 1H NMR, IR, and Mass spectral data .Molecular Structure Analysis
Esmolol-d7 Hydrochloride has a molecular formula of C16H18D7NO4 . It is the deuterium labeled Esmolol hydrochloride .Chemical Reactions Analysis
Esmolol-d7 Hydrochloride is intended for use as an internal standard for the quantification of esmolol . It binds to β1-ARs and is 34-fold selective for β1- over β2-ARs .Physical And Chemical Properties Analysis
Esmolol-d7 Hydrochloride has a molecular weight of 338.88 g/mol . It has a hydrogen bond donor count of 3 and a hydrogen bond acceptor count of 5 .Wissenschaftliche Forschungsanwendungen
Internal Standard for Quantification
Esmolol-d7 Hydrochloride is used as an internal standard for the quantification of esmolol . This is done using Gas Chromatography (GC) or Liquid Chromatography (LC) coupled with Mass Spectrometry (MS) .
β1-Adrenergic Receptor Antagonist
Esmolol-d7 Hydrochloride acts as a β1-adrenergic receptor (β1-AR) antagonist . It binds to β1-ARs and is 34-fold selective for β1- over β2-ARs .
Inhibition of L-type Ca2+ Currents and Fast Na+ Current
Esmolol-d7 Hydrochloride inhibits L-type Ca2+ currents (ICa.L) and the fast Na+ current (INa) in rat cardiac myocytes . This results in complete ventricular arrest at concentrations greater than or equal to 1 mM .
Treatment of Cardiac Arrhythmias
Formulations containing Esmolol-d7 Hydrochloride have been used in the treatment of cardiac arrhythmias . It helps in regulating the heart rate and rhythm.
Management of Postoperative Hypertension
Esmolol-d7 Hydrochloride is used in the management of postoperative hypertension . It helps in controlling high blood pressure after surgery.
Treatment of Acute Ischemic Heart Disease
Esmolol-d7 Hydrochloride is used in the treatment of acute ischemic heart disease . It helps in reducing the workload on the heart and improves blood flow.
Minimizing Myocardial Contraction during Cardiac Surgery
Esmolol-d7 Hydrochloride is used to minimize myocardial contraction during cardiac surgery . It helps in reducing the stress on the heart during surgery.
Attenuating the Adrenergic Response associated with Tracheal Intubation
Esmolol-d7 Hydrochloride is used to attenuate the adrenergic response associated with tracheal intubation . It helps in managing the physiological response during intubation.
Safety And Hazards
Zukünftige Richtungen
Esmolol is used in various settings, including urgent care, perioperative care, and postoperative care . It is indicated in sinus tachycardia, where a rapid rate requires intervention secondary to other comorbidities . Esmolol is FDA-approved for tachycardia and hypertension induced by intubation . It is used off-label for managing rate and rhythm in conditions such as aortic dissection, acute coronary syndrome, non-ST elevation myocardial infarction, hypertensive emergencies, thyrotoxicosis, refractory ventricular tachycardia, refractory to defibrillation ventricular fibrillation, and to decrease catecholamine response during electroconvulsive therapy .
Eigenschaften
IUPAC Name |
methyl 3-[4-[3-(1,1,1,2,3,3,3-heptadeuteriopropan-2-ylamino)-2-hydroxypropoxy]phenyl]propanoate;hydrochloride | |
---|---|---|
Source | PubChem | |
URL | https://pubchem.ncbi.nlm.nih.gov | |
Description | Data deposited in or computed by PubChem | |
InChI |
InChI=1S/C16H25NO4.ClH/c1-12(2)17-10-14(18)11-21-15-7-4-13(5-8-15)6-9-16(19)20-3;/h4-5,7-8,12,14,17-18H,6,9-11H2,1-3H3;1H/i1D3,2D3,12D; | |
Source | PubChem | |
URL | https://pubchem.ncbi.nlm.nih.gov | |
Description | Data deposited in or computed by PubChem | |
InChI Key |
GEKNCWBANDDJJL-ODLOEXKQSA-N | |
Source | PubChem | |
URL | https://pubchem.ncbi.nlm.nih.gov | |
Description | Data deposited in or computed by PubChem | |
Canonical SMILES |
CC(C)NCC(COC1=CC=C(C=C1)CCC(=O)OC)O.Cl | |
Source | PubChem | |
URL | https://pubchem.ncbi.nlm.nih.gov | |
Description | Data deposited in or computed by PubChem | |
Isomeric SMILES |
[2H]C([2H])([2H])C([2H])(C([2H])([2H])[2H])NCC(COC1=CC=C(C=C1)CCC(=O)OC)O.Cl | |
Source | PubChem | |
URL | https://pubchem.ncbi.nlm.nih.gov | |
Description | Data deposited in or computed by PubChem | |
Molecular Formula |
C16H26ClNO4 | |
Source | PubChem | |
URL | https://pubchem.ncbi.nlm.nih.gov | |
Description | Data deposited in or computed by PubChem | |
Molecular Weight |
338.88 g/mol | |
Source | PubChem | |
URL | https://pubchem.ncbi.nlm.nih.gov | |
Description | Data deposited in or computed by PubChem | |
Product Name |
Esmolol-d7 Hydrochloride |
Retrosynthesis Analysis
AI-Powered Synthesis Planning: Our tool employs the Template_relevance Pistachio, Template_relevance Bkms_metabolic, Template_relevance Pistachio_ringbreaker, Template_relevance Reaxys, Template_relevance Reaxys_biocatalysis model, leveraging a vast database of chemical reactions to predict feasible synthetic routes.
One-Step Synthesis Focus: Specifically designed for one-step synthesis, it provides concise and direct routes for your target compounds, streamlining the synthesis process.
Accurate Predictions: Utilizing the extensive PISTACHIO, BKMS_METABOLIC, PISTACHIO_RINGBREAKER, REAXYS, REAXYS_BIOCATALYSIS database, our tool offers high-accuracy predictions, reflecting the latest in chemical research and data.
Strategy Settings
Precursor scoring | Relevance Heuristic |
---|---|
Min. plausibility | 0.01 |
Model | Template_relevance |
Template Set | Pistachio/Bkms_metabolic/Pistachio_ringbreaker/Reaxys/Reaxys_biocatalysis |
Top-N result to add to graph | 6 |
Feasible Synthetic Routes
Haftungsausschluss und Informationen zu In-Vitro-Forschungsprodukten
Bitte beachten Sie, dass alle Artikel und Produktinformationen, die auf BenchChem präsentiert werden, ausschließlich zu Informationszwecken bestimmt sind. Die auf BenchChem zum Kauf angebotenen Produkte sind speziell für In-vitro-Studien konzipiert, die außerhalb lebender Organismen durchgeführt werden. In-vitro-Studien, abgeleitet von dem lateinischen Begriff "in Glas", beinhalten Experimente, die in kontrollierten Laborumgebungen unter Verwendung von Zellen oder Geweben durchgeführt werden. Es ist wichtig zu beachten, dass diese Produkte nicht als Arzneimittel oder Medikamente eingestuft sind und keine Zulassung der FDA für die Vorbeugung, Behandlung oder Heilung von medizinischen Zuständen, Beschwerden oder Krankheiten erhalten haben. Wir müssen betonen, dass jede Form der körperlichen Einführung dieser Produkte in Menschen oder Tiere gesetzlich strikt untersagt ist. Es ist unerlässlich, sich an diese Richtlinien zu halten, um die Einhaltung rechtlicher und ethischer Standards in Forschung und Experiment zu gewährleisten.