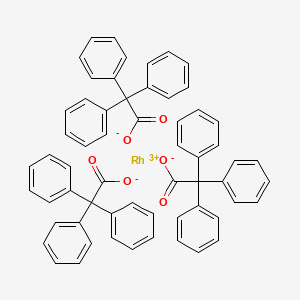
Rhodiumtriphenylacetat
- Klicken Sie auf QUICK INQUIRY, um ein Angebot von unserem Expertenteam zu erhalten.
- Mit qualitativ hochwertigen Produkten zu einem WETTBEWERBSFÄHIGEN Preis können Sie sich mehr auf Ihre Forschung konzentrieren.
Übersicht
Beschreibung
Rhodium triphenyl acetate is an organometallic compound that features rhodium as the central metal atom coordinated with triphenyl acetate ligands. This compound is known for its applications in catalysis, particularly in organic synthesis and industrial processes. It is a versatile reagent and catalyst, often used in thin film deposition, industrial chemistry, pharmaceuticals, and LED manufacturing .
Wissenschaftliche Forschungsanwendungen
Rhodium triphenyl acetate has a wide range of applications in scientific research, including:
Chemistry: It is used as a catalyst in various organic reactions, such as hydroformylation and hydrogenation.
Biology: The compound is studied for its potential biological activity and interactions with biomolecules.
Medicine: Research is ongoing to explore its potential use in medicinal chemistry, particularly in the development of new drugs.
Wirkmechanismus
Target of Action
Rhodium triphenyl acetate, also known as rhodium(3+);2,2,2-triphenylacetate, is primarily used as a catalyst in various organic transformations . The primary targets of this compound are the reactant molecules in these transformations. The role of rhodium triphenyl acetate is to accelerate the reaction rate and increase the yield of the desired product .
Mode of Action
The mode of action of rhodium triphenyl acetate involves its interaction with reactant molecules to facilitate their transformation. It is suggested that rhodium-centered radical species, generated from various tertiary phosphines via phosphine–oxidant charge transfer processes, photoredox catalysis, and electrochemical oxidations, can give rise to many unprecedented activation modes and reactions .
Biochemical Pathways
These transformations can involve a variety of biochemical pathways, depending on the specific reactants and conditions .
Result of Action
The result of rhodium triphenyl acetate’s action is the facilitation of various organic transformations. These transformations can lead to the formation of a wide range of organic compounds, including cyclic carbamates, nitrene insertions, and cyclic oxonium ylides .
Vorbereitungsmethoden
Synthetic Routes and Reaction Conditions: Rhodium triphenyl acetate can be synthesized through the reaction of rhodium chloride with triphenyl acetate in the presence of a suitable base. The reaction typically involves heating the mixture under reflux conditions to facilitate the formation of the desired complex. The product is then purified through recrystallization or other suitable methods .
Industrial Production Methods: In industrial settings, the production of rhodium triphenyl acetate involves large-scale reactions under controlled conditions to ensure high yield and purity. The process may include the use of automated reactors and purification systems to streamline the production and maintain consistency in the quality of the compound .
Analyse Chemischer Reaktionen
Types of Reactions: Rhodium triphenyl acetate undergoes various types of chemical reactions, including:
Oxidation: The compound can be oxidized to form rhodium(III) species.
Reduction: It can be reduced back to rhodium(I) species under suitable conditions.
Substitution: The triphenyl acetate ligands can be substituted with other ligands, depending on the reaction conditions and reagents used.
Common Reagents and Conditions:
Oxidation: Common oxidizing agents include oxygen and peroxides.
Reduction: Reducing agents such as hydrogen gas or hydrides are used.
Substitution: Ligand exchange reactions often involve phosphines, amines, or other coordinating ligands.
Major Products Formed: The major products formed from these reactions depend on the specific reagents and conditions used. For example, oxidation may yield rhodium(III) complexes, while reduction can regenerate rhodium(I) species. Substitution reactions can produce a variety of rhodium complexes with different ligands .
Vergleich Mit ähnlichen Verbindungen
Rhodium triphenyl phosphine: Another rhodium complex with triphenyl phosphine ligands, used in similar catalytic applications.
Rhodium acetate: A simpler rhodium complex with acetate ligands, also used in catalysis.
Rhodium carbonyl complexes: These complexes feature carbonyl ligands and are used in hydroformylation and other catalytic processes.
Uniqueness: Rhodium triphenyl acetate is unique due to the presence of triphenyl acetate ligands, which provide distinct steric and electronic properties. This uniqueness allows for specific catalytic activities and selectivities that may not be achievable with other rhodium complexes. The compound’s versatility and stability make it a valuable reagent in both research and industrial applications .
Biologische Aktivität
Rhodium triphenyl acetate (RTA), a coordination compound of rhodium, has garnered attention in various fields, particularly in organic chemistry and medicinal applications. This article delves into the biological activity of RTA, exploring its mechanisms of action, biochemical pathways, and potential therapeutic applications.
Rhodium triphenyl acetate is primarily utilized as a catalyst in organic transformations. Its biological activity is attributed to its ability to interact with biomolecules, facilitating various chemical reactions. The compound can generate rhodium-centered radical species through mechanisms such as photoredox catalysis and charge transfer processes involving tertiary phosphines.
Key Mechanisms:
- Catalytic Action: RTA acts as a catalyst in reactions like hydroformylation and hydrogenation, enhancing the formation of organic compounds.
- Radical Generation: The formation of rhodium radicals allows for unprecedented activation modes, which can lead to diverse biochemical transformations .
Biochemical Pathways
The interactions of RTA with biological systems can lead to various biochemical pathways:
- Organic Transformations: RTA facilitates the synthesis of cyclic carbamates and nitrene insertions, which are crucial in drug development and material science.
- Metabolic Pathways: Studies have shown that rhodium compounds may influence metabolic processes in tumor-bearing models, highlighting their potential role in cancer therapeutics .
Biological Activity and Therapeutic Potential
Research into the biological activity of RTA indicates its potential as a therapeutic agent. Some notable findings include:
- Antitumor Activity: In vivo studies have demonstrated that rhodium acetate derivatives can be incorporated into tumor cells, suggesting a possible mechanism for targeting cancer cells .
- Drug Development: Ongoing research is examining RTA's role in medicinal chemistry, aiming to develop new drugs that leverage its catalytic properties for therapeutic purposes .
Case Studies
Several studies have investigated the biological effects of rhodium complexes, including RTA:
- Ehrlich Ascites Tumor Model: A study assessed the metabolism of rhodium(II) acetate in tumor-bearing mice, revealing rapid distribution and excretion patterns. The liver was identified as the primary organ for rhodium deposition, with minimal amounts detected in brain tissue .
- Catalytic Reactions: Research has shown that RTA can effectively catalyze the transannulation of triazoles with nitriles, yielding imidazoles with high selectivity. This reaction underscores the compound's utility in organic synthesis and potential pharmaceutical applications .
Data Table: Summary of Biological Activities
Eigenschaften
IUPAC Name |
rhodium(3+);2,2,2-triphenylacetate |
Source
|
---|---|---|
Source | PubChem | |
URL | https://pubchem.ncbi.nlm.nih.gov | |
Description | Data deposited in or computed by PubChem | |
InChI |
InChI=1S/3C20H16O2.Rh/c3*21-19(22)20(16-10-4-1-5-11-16,17-12-6-2-7-13-17)18-14-8-3-9-15-18;/h3*1-15H,(H,21,22);/q;;;+3/p-3 |
Source
|
Source | PubChem | |
URL | https://pubchem.ncbi.nlm.nih.gov | |
Description | Data deposited in or computed by PubChem | |
InChI Key |
LJIVYHNRWSWCRU-UHFFFAOYSA-K |
Source
|
Source | PubChem | |
URL | https://pubchem.ncbi.nlm.nih.gov | |
Description | Data deposited in or computed by PubChem | |
Canonical SMILES |
C1=CC=C(C=C1)C(C2=CC=CC=C2)(C3=CC=CC=C3)C(=O)[O-].C1=CC=C(C=C1)C(C2=CC=CC=C2)(C3=CC=CC=C3)C(=O)[O-].C1=CC=C(C=C1)C(C2=CC=CC=C2)(C3=CC=CC=C3)C(=O)[O-].[Rh+3] |
Source
|
Source | PubChem | |
URL | https://pubchem.ncbi.nlm.nih.gov | |
Description | Data deposited in or computed by PubChem | |
Molecular Formula |
C60H45O6Rh |
Source
|
Source | PubChem | |
URL | https://pubchem.ncbi.nlm.nih.gov | |
Description | Data deposited in or computed by PubChem | |
Molecular Weight |
964.9 g/mol |
Source
|
Source | PubChem | |
URL | https://pubchem.ncbi.nlm.nih.gov | |
Description | Data deposited in or computed by PubChem | |
Haftungsausschluss und Informationen zu In-Vitro-Forschungsprodukten
Bitte beachten Sie, dass alle Artikel und Produktinformationen, die auf BenchChem präsentiert werden, ausschließlich zu Informationszwecken bestimmt sind. Die auf BenchChem zum Kauf angebotenen Produkte sind speziell für In-vitro-Studien konzipiert, die außerhalb lebender Organismen durchgeführt werden. In-vitro-Studien, abgeleitet von dem lateinischen Begriff "in Glas", beinhalten Experimente, die in kontrollierten Laborumgebungen unter Verwendung von Zellen oder Geweben durchgeführt werden. Es ist wichtig zu beachten, dass diese Produkte nicht als Arzneimittel oder Medikamente eingestuft sind und keine Zulassung der FDA für die Vorbeugung, Behandlung oder Heilung von medizinischen Zuständen, Beschwerden oder Krankheiten erhalten haben. Wir müssen betonen, dass jede Form der körperlichen Einführung dieser Produkte in Menschen oder Tiere gesetzlich strikt untersagt ist. Es ist unerlässlich, sich an diese Richtlinien zu halten, um die Einhaltung rechtlicher und ethischer Standards in Forschung und Experiment zu gewährleisten.