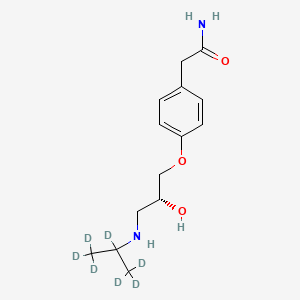
(R)-Atenolol-d7
- Klicken Sie auf QUICK INQUIRY, um ein Angebot von unserem Expertenteam zu erhalten.
- Mit qualitativ hochwertigen Produkten zu einem WETTBEWERBSFÄHIGEN Preis können Sie sich mehr auf Ihre Forschung konzentrieren.
Übersicht
Beschreibung
®-Atenolol-d7 is a deuterated form of ®-Atenolol, a beta-blocker used primarily in the treatment of cardiovascular diseases such as hypertension and angina. The deuterium atoms in ®-Atenolol-d7 replace the hydrogen atoms, which can provide insights into the metabolic pathways and pharmacokinetics of the drug.
Wissenschaftliche Forschungsanwendungen
®-Atenolol-d7 has a wide range of applications in scientific research, including:
Chemistry: Used as a tracer in studies involving metabolic pathways and reaction mechanisms.
Biology: Helps in understanding the biological processes and interactions of beta-blockers.
Medicine: Used in pharmacokinetic studies to investigate the absorption, distribution, metabolism, and excretion of beta-blockers.
Industry: Employed in the development of new pharmaceuticals and in quality control processes.
Vorbereitungsmethoden
Synthetic Routes and Reaction Conditions: The synthesis of ®-Atenolol-d7 involves the incorporation of deuterium atoms into the ®-Atenolol molecule. This can be achieved through various methods, including:
Hydrogen-Deuterium Exchange: This method involves the replacement of hydrogen atoms with deuterium in the presence of a deuterium source such as deuterium oxide (D2O) under specific reaction conditions.
Deuterated Reagents: Using deuterated reagents in the synthesis process can also introduce deuterium atoms into the molecule.
Industrial Production Methods: Industrial production of ®-Atenolol-d7 typically involves large-scale synthesis using deuterated reagents and optimized reaction conditions to ensure high yield and purity. The process may include steps such as:
Catalytic Hydrogenation: Using deuterium gas (D2) in the presence of a catalyst to replace hydrogen atoms with deuterium.
Purification: Techniques such as crystallization, distillation, and chromatography are employed to purify the final product.
Analyse Chemischer Reaktionen
Types of Reactions: ®-Atenolol-d7 undergoes various chemical reactions, including:
Oxidation: The compound can be oxidized to form corresponding oxides.
Reduction: Reduction reactions can convert ®-Atenolol-d7 into its reduced forms.
Substitution: Substitution reactions can replace functional groups within the molecule.
Common Reagents and Conditions:
Oxidizing Agents: Potassium permanganate (KMnO4), hydrogen peroxide (H2O2).
Reducing Agents: Sodium borohydride (NaBH4), lithium aluminum hydride (LiAlH4).
Substitution Reagents: Halogens, alkylating agents.
Major Products: The major products formed from these reactions depend on the specific reagents and conditions used. For example, oxidation may yield oxides, while reduction may produce alcohols or amines.
Wirkmechanismus
®-Atenolol-d7 exerts its effects by blocking beta-adrenergic receptors, which are involved in the regulation of heart rate and blood pressure. The deuterium atoms do not significantly alter the mechanism of action but can provide valuable information on the drug’s metabolic stability and pathways. The molecular targets include beta-1 adrenergic receptors, and the pathways involved are related to the inhibition of adrenergic signaling.
Vergleich Mit ähnlichen Verbindungen
®-Atenolol: The non-deuterated form of the compound.
(S)-Atenolol: The enantiomer of ®-Atenolol.
Metoprolol: Another beta-blocker with similar therapeutic uses.
Uniqueness: ®-Atenolol-d7 is unique due to the presence of deuterium atoms, which can provide insights into the drug’s pharmacokinetics and metabolic pathways. This makes it a valuable tool in research and development, offering advantages in studies requiring precise tracking and analysis of the compound.
Biologische Aktivität
(R)-Atenolol-d7 is a deuterated form of atenolol, a selective beta-1 adrenergic blocker primarily used in the treatment of hypertension and cardiovascular diseases. The incorporation of deuterium atoms in this compound allows for enhanced pharmacokinetic studies and serves as an internal standard in analytical chemistry. This article will explore the biological activity of this compound, including its pharmacokinetics, metabolic pathways, and implications in clinical research.
Pharmacokinetics of this compound
Pharmacokinetics refers to how a drug is absorbed, distributed, metabolized, and excreted in the body. The unique properties of this compound influence its pharmacokinetic profile.
Absorption and Distribution
- Oral Bioavailability : Atenolol exhibits low passive permeability due to its hydrophilic nature, which affects its absorption in the gastrointestinal tract. Studies have shown that atenolol's absorption can be influenced by various factors such as gastric pH and the presence of food .
- Blood-Brain Barrier (BBB) Penetration : Research indicates that this compound can be used to study the transport mechanisms across the BBB. Its low unbound equilibrium partition coefficient suggests limited penetration into brain tissue, making it a useful marker for assessing BBB permeability .
Metabolism
- Renal Clearance : Atenolol is primarily eliminated through renal pathways. Studies indicate that renal secretion is mediated by organic cation transporters, which play a crucial role in the drug's pharmacokinetics . The metabolic stability of this compound allows for precise measurements in pharmacokinetic studies.
- Biotransformation : Atenolol undergoes biotransformation to form various metabolites, with atenolol acid being one of the significant products. This transformation can be influenced by enzymatic activity related to ammonium oxidation .
Case Studies
Several case studies have highlighted the biological activity and clinical implications of this compound:
- Study on Metabolic Effects : A clinical trial involving patients on atenolol therapy revealed that increased exposure to atenolol correlated with adverse metabolic effects, such as alterations in fasting glucose levels. This study utilized this compound as an internal standard to ensure accurate plasma concentration measurements .
- BBB Transport Mechanisms : In an experimental setup using microdialysis, this compound was employed as a calibrator to assess the relative recovery of atenolol across brain microdialysis probes. The findings indicated that while systemic absorption was significant, BBB transport was limited due to active efflux mechanisms .
Research Findings
The following table summarizes key research findings related to the biological activity of this compound:
Eigenschaften
IUPAC Name |
2-[4-[(2R)-3-(1,1,1,2,3,3,3-heptadeuteriopropan-2-ylamino)-2-hydroxypropoxy]phenyl]acetamide |
Source
|
---|---|---|
Source | PubChem | |
URL | https://pubchem.ncbi.nlm.nih.gov | |
Description | Data deposited in or computed by PubChem | |
InChI |
InChI=1S/C14H22N2O3/c1-10(2)16-8-12(17)9-19-13-5-3-11(4-6-13)7-14(15)18/h3-6,10,12,16-17H,7-9H2,1-2H3,(H2,15,18)/t12-/m1/s1/i1D3,2D3,10D |
Source
|
Source | PubChem | |
URL | https://pubchem.ncbi.nlm.nih.gov | |
Description | Data deposited in or computed by PubChem | |
InChI Key |
METKIMKYRPQLGS-FSJJVVIYSA-N |
Source
|
Source | PubChem | |
URL | https://pubchem.ncbi.nlm.nih.gov | |
Description | Data deposited in or computed by PubChem | |
Canonical SMILES |
CC(C)NCC(COC1=CC=C(C=C1)CC(=O)N)O |
Source
|
Source | PubChem | |
URL | https://pubchem.ncbi.nlm.nih.gov | |
Description | Data deposited in or computed by PubChem | |
Isomeric SMILES |
[2H]C([2H])([2H])C([2H])(C([2H])([2H])[2H])NC[C@H](COC1=CC=C(C=C1)CC(=O)N)O |
Source
|
Source | PubChem | |
URL | https://pubchem.ncbi.nlm.nih.gov | |
Description | Data deposited in or computed by PubChem | |
Molecular Formula |
C14H22N2O3 |
Source
|
Source | PubChem | |
URL | https://pubchem.ncbi.nlm.nih.gov | |
Description | Data deposited in or computed by PubChem | |
Molecular Weight |
273.38 g/mol |
Source
|
Source | PubChem | |
URL | https://pubchem.ncbi.nlm.nih.gov | |
Description | Data deposited in or computed by PubChem | |
Haftungsausschluss und Informationen zu In-Vitro-Forschungsprodukten
Bitte beachten Sie, dass alle Artikel und Produktinformationen, die auf BenchChem präsentiert werden, ausschließlich zu Informationszwecken bestimmt sind. Die auf BenchChem zum Kauf angebotenen Produkte sind speziell für In-vitro-Studien konzipiert, die außerhalb lebender Organismen durchgeführt werden. In-vitro-Studien, abgeleitet von dem lateinischen Begriff "in Glas", beinhalten Experimente, die in kontrollierten Laborumgebungen unter Verwendung von Zellen oder Geweben durchgeführt werden. Es ist wichtig zu beachten, dass diese Produkte nicht als Arzneimittel oder Medikamente eingestuft sind und keine Zulassung der FDA für die Vorbeugung, Behandlung oder Heilung von medizinischen Zuständen, Beschwerden oder Krankheiten erhalten haben. Wir müssen betonen, dass jede Form der körperlichen Einführung dieser Produkte in Menschen oder Tiere gesetzlich strikt untersagt ist. Es ist unerlässlich, sich an diese Richtlinien zu halten, um die Einhaltung rechtlicher und ethischer Standards in Forschung und Experiment zu gewährleisten.