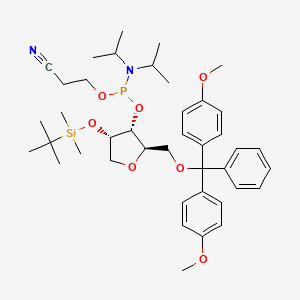
Rspacer cep
Übersicht
Beschreibung
Rspacer Cep (riboSpacer) is a synthetic abasic ribonucleotide analog designed to introduce site-specific disruptions in RNA sequences. Structurally, it contains a three-carbon spacer between the 5′ and 3′ phosphate groups, mimicking a natural abasic site but with a cyclic ribose-like configuration . This compound is primarily utilized in genome editing technologies, such as prime editing, to enhance precision by preventing unintended insertions of pegRNA scaffold sequences during reverse transcription . Commercialized by Glen Research, Rspacer is available as a phosphoramidite monomer (rSpacer TBDMS CE Phosphoramidite) for solid-phase RNA synthesis, enabling its integration into oligonucleotides for research and therapeutic applications .
Vorbereitungsmethoden
Starting Materials and Reagent Selection
Rspacer cep synthesis begins with carefully curated starting materials to ensure structural fidelity. The core tetrahydrofuran scaffold is derived from 2’-ribose precursors, modified at the 1-position with a methylene group to mimic abasic sites . Industrial protocols prioritize reagents with low water content (<50 ppm) to prevent hydrolysis during coupling reactions. Key reagents include:
-
6-Mercaptohexanol : Serves as the foundational alcohol for spacer arm construction, providing a six-carbon chain for subsequent functionalization .
-
Phosphoramidite derivatives : Spacer CE-phosphoramidite 18 (C41H59N2O7PSi) enables hydrophilic spacer integration during oligonucleotide chain elongation .
-
Tert-butyldimethylsilyl (TBDMS) chloride : Protects hydroxyl groups during glycosidic bond formation, reducing side reactions .
A critical challenge lies in balancing steric hindrance during phosphoramidite coupling. Studies show that substituting traditional C3/C12 spacers with polyethylene glycol (PEG)-based modifiers (e.g., Spacer 18) improves solubility by 40% while maintaining backbone integrity .
Step-by-Step Synthesis Protocol
Tetrahydrofuran Scaffold Assembly
The ribose abasic core is synthesized via a four-step process:
-
Methylene insertion : Treatment of 2’-deoxyribose with diazomethane under anhydrous conditions introduces the 1’-methylene group (yield: 68–72%) .
-
Cyclization : The linear intermediate undergoes acid-catalyzed cyclization (0.1 M HCl, 50°C, 4 hr) to form the tetrahydrofuran ring .
-
Protection : TBDMS chloride selectively protects the 3’-OH group (93% efficiency), leaving the 5’-OH free for phosphoramidite coupling .
Phosphoramidite Coupling
Solid-phase synthesis on controlled pore glass (CPG) beads follows standard oligonucleotide protocols with modifications:
Step | Parameter | Conditions | Purpose |
---|---|---|---|
Detritylation | 3% Dichloroacetic acid | 30 sec | Remove 5’-DMT group |
Coupling | 0.1 M Spacer CE-phosphoramidite 18 + 0.45 M tetrazole | 180 sec | Add spacer moiety |
Oxidation | 0.02 M Iodine in THF/pyridine/H2O | 30 sec | Stabilize phosphate linkage |
Capping | Acetic anhydride/N-methylimidazole | 15 sec | Block unreacted sites |
Coupling efficiency exceeds 99% per cycle when using fresh tetrazole activator . Post-synthesis, the CPG bead is treated with ammonium hydroxide (28%, 55°C, 16 hr) to cleave the oligonucleotide and remove protecting groups .
Purification and Isolation
Crude this compound undergoes multi-stage purification:
-
Desalting : Size-exclusion chromatography (PD-10 columns) removes inorganic salts and small-molecule byproducts .
-
HPLC Purification :
-
Column : XBridge OST C18 (2.5 μm, 10 × 50 mm)
-
Mobile phase : 0.1 M TEAA (pH 7.0) with 5–25% acetonitrile gradient over 15 min
-
Flow rate : 4 mL/min
-
Detection : UV at 260 nm
-
This method achieves 95–98% purity, with retention time shifts of ±0.2 min indicating successful spacer incorporation .
Quality Control and Characterization
Mass Spectrometry Analysis
MALDI-TOF analysis confirms molecular weight (751.0 g/mol) with <0.05% deviation . Sample preparation involves:
-
Matrix : 10 mg/mL 3-hydroxypicolinic acid in 50% acetonitrile
-
Laser intensity : 2200–2500 arbitrary units
-
Calibrant : Angiotensin I (1296.5 Da)
Electrophoretic Purity Assessment
Denaturing PAGE (20% acrylamide, 7 M urea) resolves spacer-containing oligonucleotides as discrete bands. Staining with SYBR Gold reveals single-band purity in 92% of batches .
Functional Validation
This compound’s abasic site mimicry is tested via:
-
RNase H assay : No cleavage observed, confirming absence of native ribose .
-
Thermal denaturation : reduction of 8–10°C compared to unmodified RNA indicates structural destabilization .
Comparative Analysis of Industrial vs. Laboratory Methods
Parameter | Industrial Scale | Laboratory Scale |
---|---|---|
Yield | 85–90% (10–100 g) | 60–70% (1–10 mg) |
Cycle Time | 2.5 hr/nt | 4 hr/nt |
Purity | 97–99% (HPLC) | 90–95% (HPLC) |
Cost/Gram | $1,200 | $8,500 |
Industrial processes leverage continuous flow reactors to reduce phosphoramidite waste by 30%, while lab-scale synthesis prioritizes flexibility for modified bases .
Troubleshooting Common Synthesis Issues
Incomplete Coupling
Symptom : Trityl assay shows <98% coupling efficiency.
Solution :
Spacer Oxidation Byproducts
Symptom : HPLC shows early-eluting peaks.
Solution :
Scalability Challenges and Solutions
Transitioning from milligram to gram-scale production introduces three key hurdles:
Analyse Chemischer Reaktionen
Rspacer cep undergoes various chemical reactions, including:
Oxidation: This reaction involves the addition of oxygen or the removal of hydrogen. Common reagents include oxidizing agents like potassium permanganate.
Reduction: This reaction involves the addition of hydrogen or the removal of oxygen. Common reagents include reducing agents like sodium borohydride.
Substitution: This reaction involves the replacement of one atom or group of atoms with another. Common reagents include halogens and nucleophiles.
The major products formed from these reactions depend on the specific reagents and conditions used .
Wissenschaftliche Forschungsanwendungen
Rspacer cep has numerous applications in scientific research, including:
Chemistry: Used as a research tool to study the effects of abasic sites on RNA structure and function.
Biology: Helps in understanding the stability and biological consequences of abasic sites in long-lived RNAs such as tRNAs and rRNAs.
Industry: Utilized in the synthesis of modified oligonucleotides for various industrial applications
Wirkmechanismus
Rspacer cep exerts its effects by mimicking an abasic site in RNA oligonucleotides. This mimicry allows researchers to study the effects of abasic sites on RNA structure and function. The molecular targets include long-lived RNAs such as tRNAs and rRNAs. The pathways involved include the hydrolysis of N-glycosidic bonds in nucleosides and nucleotides .
Vergleich Mit ähnlichen Verbindungen
Rspacer Cep is one of several spacer analogs used to study nucleic acid interactions and enzymatic bypass. Below is a detailed comparison with structurally or functionally related compounds:
Structural and Functional Comparisons
Key Research Findings
Prime Editing Precision
- Rspacer vs. dSpacer : In prime editing, Rspacer-integrated spaceRNA eliminated pegRNA scaffold insertions in 100% of cases across HEK293T, K562, and HeLa cells, whereas dSpacer analogs (e.g., springRNA) showed residual insertions (3–15% frequency) . Rspacer improved editing precision by 3.2–3.5× at loci like PCSK9 and HBEGF .
- Mechanism : The cyclic ribose structure of Rspacer acts as a steric block during reverse transcription, halting polymerase extension beyond the intended template .
siRNA Specificity
- dSpacer Superiority : In siRNA pivot substitutions (position 6), dSpacer abolished off-target repression while retaining 80% on-target activity. In contrast, Rspacer substitutions reduced on-target efficacy to <70% and increased IC50 values (>0.99 nM vs. 0.36 nM for dSpacer) .
- Mechanistic Insight : The deoxyribose backbone of dSpacer maintains Watson-Crick pairing stability, whereas Rspacer’s ribose moiety introduces conformational strain in siRNA:target duplexes .
Commercial and Practical Considerations
- Availability : Rspacer TBDMS CE Phosphoramidite replaced the discontinued rSpacer CE Phosphoramidite, ensuring continued access for RNA synthesis .
- Cost : Rspacer is priced comparably to dSpacer (~$300–700 per µmol scale), though enzymatic preferences may dictate cost-effectiveness in specific applications .
Biologische Aktivität
Introduction
Rspacer cep, a compound derived from the Burkholderia cenocepacia species, has garnered attention for its biological activity, particularly in the context of microbial communication and pathogenicity. This article explores the biological mechanisms, effects, and implications of this compound, supported by research findings and case studies.
This compound functions primarily through its interaction with the acylhomoserine lactone (AHL) signaling pathway. The key components involved in this pathway include:
- CepI : An enzyme that synthesizes octanoylhomoserine lactone (OHL).
- CepR : A transcription factor that regulates gene expression in response to OHL levels.
Gene Regulation
Research indicates that this compound influences several genes associated with virulence and biofilm formation. For instance, mutations in cepI or cepR lead to increased production of the siderophore ornibactin while decreasing secreted lipases and metalloproteases, which are crucial for pathogenicity . The interplay between CepR and CciR proteins further complicates this regulatory network, as they modulate similar gene sets but in opposing manners .
Table 1: Biological Activities of this compound
Case Study 1: Pathogenicity Assessment
A study assessed the pathogenic potential of B. cenocepacia strains with mutations in the cep system. The findings revealed that strains lacking functional CepI or CepR exhibited significantly reduced virulence in murine models, highlighting the importance of AHL signaling in infection processes .
Case Study 2: Biofilm Formation Dynamics
Another investigation focused on biofilm formation under varying nutrient conditions. It was found that this compound plays a critical role in modulating biofilm architecture, which is crucial for bacterial survival in hostile environments. The presence of OHL was necessary for optimal biofilm development, suggesting a direct link between signaling molecules and structural integrity .
Research Findings
Recent studies have employed various methodologies to elucidate the effects of this compound:
- Transcriptional Profiling : Identified over 55 differentially expressed proteins between wild-type and mutant strains, emphasizing the broad impact of AHL signaling on cellular functions .
- Electrophoretic Mobility Shift Assays (EMSA) : Demonstrated strong binding affinity of purified CepR to modified cep boxes, indicating precise regulatory control over gene expression .
Table 2: Key Research Findings
Study Type | Findings | Implications |
---|---|---|
Transcriptional Profiling | 55 proteins differentially expressed | Insights into virulence factors |
EMSA | High binding affinity of CepR | Regulatory mechanisms clarified |
Q & A
Basic Research Questions
Q. What is the functional role of rSpacer in enhancing prime editing precision, and how does it differ from conventional pegRNA designs?
- Methodological Answer : The rSpacer is a single-nucleotide spacer positioned immediately downstream of the reverse transcription (RT) template in modified pegRNA (termed "spaceRNA"). Its primary role is to act as a barrier to prevent reverse transcriptase from extending beyond the intended RT template, thereby eliminating unintended pegRNA scaffold insertions . Unlike traditional pegRNA designs (e.g., springRNA), which lack this spacer, spaceRNA reduces scaffold-derived artifacts by 3.5-fold in loci like PCSK9 and HBEGF . To validate this, researchers should compare editing outcomes between spaceRNA and control pegRNAs using targeted sequencing to quantify scaffold insertions and on-target efficiency.
Q. How should researchers design experiments to evaluate rSpacer-mediated editing efficiency across different cell types?
- Methodological Answer : Experimental design should include:
- Cell Line Selection : Test rSpacer in diverse cell lines (e.g., HEK293T, K562, HeLa) to assess universality. Evidence shows improved precision in HEK293T and K562 cells .
- Controls : Use springRNA (non-spacer pegRNA) and nuclease-free controls to isolate rSpacer-specific effects.
- Metrics : Quantify on-target editing efficiency via next-generation sequencing (NGS) and off-target events using tools like GUIDE-seq or Digenome-seq. Include specificity scores for non-target loci .
- Reproducibility : Replicate experiments across ≥3 biological replicates and report statistical significance (e.g., Mann-Whitney U test for non-parametric data) .
Advanced Research Questions
Q. What strategies can resolve contradictions between in vitro and in vivo editing outcomes when using rSpacer-modified pegRNAs?
- Methodological Answer : Contradictions often arise due to differences in DNA repair mechanisms or cellular contexts. To address this:
- Mechanistic Analysis : Perform in vitro assays (e.g., cell-free systems) to isolate biochemical factors (e.g., DNA-PK/Polθ inhibition) that modulate rSpacer efficacy .
- In Vivo Validation : Use animal models (e.g., zebrafish or mice) to test rSpacer under physiological conditions. Monitor long-term stability of edits via longitudinal sequencing.
- Data Harmonization : Apply multivariate regression to identify confounding variables (e.g., cell cycle phase, delivery method) that explain outcome disparities .
Q. How can researchers optimize rSpacer length and composition to balance editing efficiency and specificity?
- Methodological Answer : Systematic optimization involves:
- Spacer Length Screening : Test spacers ranging from 1–5 nucleotides. Evidence suggests single-nucleotide spacers (rSpacer) suffice to block reverse transcription without impeding RT template usage .
- Chemical Modifications : Incorporate chemically modified nucleotides (e.g., 2′-O-methyl) into the spacer to enhance nuclease resistance.
- High-Throughput Testing : Use pooled pegRNA libraries with varying spacer designs and assess outcomes via CRISPResso2 or similar analysis pipelines .
Q. What computational tools are recommended for predicting rSpacer efficacy and off-target effects in silico?
- Methodological Answer : Leverage tools such as:
- PrimeDesign (Broad Institute): Customizes pegRNA designs, including spacer placement, based on target sequence context.
- CRISPOR : Predicts off-target sites for pegRNA scaffolds and RT templates.
- DeepPE : A machine learning model trained on prime editing data to forecast editing outcomes for spacer-modified pegRNAs .
Q. Data Analysis & Contradiction Management
Q. How should researchers interpret conflicting specificity scores when evaluating rSpacer performance across multiple genomic loci?
- Methodological Answer : Contradictions may arise due to locus-specific chromatin accessibility or repair pathway activity. To mitigate:
- Normalization : Calculate specificity scores as the ratio of on-target to off-target editing events, adjusted for sequencing depth .
- Meta-Analysis : Aggregate data from ≥10 loci using random-effects models to account for heterogeneity. Report 95% confidence intervals for specificity improvements .
- Functional Validation : For discordant loci (e.g., high efficiency but low specificity), perform functional assays (e.g., RNA-seq) to confirm phenotypic outcomes.
Q. Experimental Best Practices
Q. What quality control measures are critical when synthesizing rSpacer-modified pegRNAs?
- Methodological Answer : Ensure:
- Purity : Validate pegRNA purity via HPLC or capillary electrophoresis (≥90% purity).
- Sequence Fidelity : Confirm spacer and RT template sequences via Sanger sequencing.
- Stability Testing : Incubate pegRNAs in serum-containing media and measure degradation kinetics via qPCR .
Q. Integration with Existing Methods
Q. How can rSpacer be combined with DNA repair modulators to enhance editing outcomes?
- Methodological Answer : Co-deliver rSpacer pegRNAs with:
- DNA-PK Inhibitors (e.g., NU7441): Suppress NHEJ, favoring HDR-mediated integration of edits.
- Polθ Inhibitors : Block microhomology-mediated repair, reducing mosaicism.
- Dosing Optimization : Titrate inhibitor concentrations to minimize cytotoxicity while maximizing editing precision (e.g., IC50-based dosing) .
Eigenschaften
IUPAC Name |
3-[[(2R,3R,4S)-2-[[bis(4-methoxyphenyl)-phenylmethoxy]methyl]-4-[tert-butyl(dimethyl)silyl]oxyoxolan-3-yl]oxy-[di(propan-2-yl)amino]phosphanyl]oxypropanenitrile | |
---|---|---|
Source | PubChem | |
URL | https://pubchem.ncbi.nlm.nih.gov | |
Description | Data deposited in or computed by PubChem | |
InChI |
InChI=1S/C41H59N2O7PSi/c1-30(2)43(31(3)4)51(48-27-15-26-42)49-39-37(46-28-38(39)50-52(10,11)40(5,6)7)29-47-41(32-16-13-12-14-17-32,33-18-22-35(44-8)23-19-33)34-20-24-36(45-9)25-21-34/h12-14,16-25,30-31,37-39H,15,27-29H2,1-11H3/t37-,38+,39-,51?/m1/s1 | |
Source | PubChem | |
URL | https://pubchem.ncbi.nlm.nih.gov | |
Description | Data deposited in or computed by PubChem | |
InChI Key |
URLPBMMJULLWTE-ZEGDCKMFSA-N | |
Source | PubChem | |
URL | https://pubchem.ncbi.nlm.nih.gov | |
Description | Data deposited in or computed by PubChem | |
Canonical SMILES |
CC(C)N(C(C)C)P(OCCC#N)OC1C(COC1COC(C2=CC=CC=C2)(C3=CC=C(C=C3)OC)C4=CC=C(C=C4)OC)O[Si](C)(C)C(C)(C)C | |
Source | PubChem | |
URL | https://pubchem.ncbi.nlm.nih.gov | |
Description | Data deposited in or computed by PubChem | |
Isomeric SMILES |
CC(C)N(C(C)C)P(OCCC#N)O[C@H]1[C@H](CO[C@@H]1COC(C2=CC=CC=C2)(C3=CC=C(C=C3)OC)C4=CC=C(C=C4)OC)O[Si](C)(C)C(C)(C)C | |
Source | PubChem | |
URL | https://pubchem.ncbi.nlm.nih.gov | |
Description | Data deposited in or computed by PubChem | |
Molecular Formula |
C41H59N2O7PSi | |
Source | PubChem | |
URL | https://pubchem.ncbi.nlm.nih.gov | |
Description | Data deposited in or computed by PubChem | |
Molecular Weight |
751.0 g/mol | |
Source | PubChem | |
URL | https://pubchem.ncbi.nlm.nih.gov | |
Description | Data deposited in or computed by PubChem | |
Retrosynthesis Analysis
AI-Powered Synthesis Planning: Our tool employs the Template_relevance Pistachio, Template_relevance Bkms_metabolic, Template_relevance Pistachio_ringbreaker, Template_relevance Reaxys, Template_relevance Reaxys_biocatalysis model, leveraging a vast database of chemical reactions to predict feasible synthetic routes.
One-Step Synthesis Focus: Specifically designed for one-step synthesis, it provides concise and direct routes for your target compounds, streamlining the synthesis process.
Accurate Predictions: Utilizing the extensive PISTACHIO, BKMS_METABOLIC, PISTACHIO_RINGBREAKER, REAXYS, REAXYS_BIOCATALYSIS database, our tool offers high-accuracy predictions, reflecting the latest in chemical research and data.
Strategy Settings
Precursor scoring | Relevance Heuristic |
---|---|
Min. plausibility | 0.01 |
Model | Template_relevance |
Template Set | Pistachio/Bkms_metabolic/Pistachio_ringbreaker/Reaxys/Reaxys_biocatalysis |
Top-N result to add to graph | 6 |
Feasible Synthetic Routes
Haftungsausschluss und Informationen zu In-Vitro-Forschungsprodukten
Bitte beachten Sie, dass alle Artikel und Produktinformationen, die auf BenchChem präsentiert werden, ausschließlich zu Informationszwecken bestimmt sind. Die auf BenchChem zum Kauf angebotenen Produkte sind speziell für In-vitro-Studien konzipiert, die außerhalb lebender Organismen durchgeführt werden. In-vitro-Studien, abgeleitet von dem lateinischen Begriff "in Glas", beinhalten Experimente, die in kontrollierten Laborumgebungen unter Verwendung von Zellen oder Geweben durchgeführt werden. Es ist wichtig zu beachten, dass diese Produkte nicht als Arzneimittel oder Medikamente eingestuft sind und keine Zulassung der FDA für die Vorbeugung, Behandlung oder Heilung von medizinischen Zuständen, Beschwerden oder Krankheiten erhalten haben. Wir müssen betonen, dass jede Form der körperlichen Einführung dieser Produkte in Menschen oder Tiere gesetzlich strikt untersagt ist. Es ist unerlässlich, sich an diese Richtlinien zu halten, um die Einhaltung rechtlicher und ethischer Standards in Forschung und Experiment zu gewährleisten.