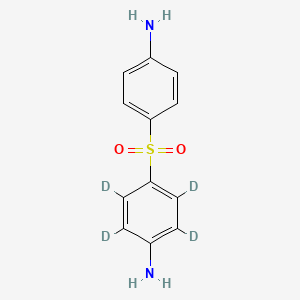
Dapsone-d4
Übersicht
Beschreibung
Dapsone D4, chemically known as 4-((4-Aminophenyl)sulfonyl)benzen-2,3,5,6-d4-amine, is used for analytical method development, method validation (AMV), and Quality Controlled (QC) application for Abbreviated New Drug Application (ANDA) or during commercial production of Dapsone .
Synthesis Analysis
Dapsone and its analogues have been synthesized and tested on established and primary cultured glioma cells to determine effects on proliferation, anchorage-independent growth, and migration . The crystal engineering method of cocrystallization has been used to study new solid forms of dapsone . A conceptual DFT analysis was carried out to gain more insight into the redox process .Molecular Structure Analysis
The chemical structure of Dapsone D4 consists of two benzene rings connected by a sulfone group (-SO2-). This structure gives Dapsone D4 its unique antimicrobial properties, as it is able to inhibit the growth of bacteria, fungi, and parasites by interfering with their ability to produce folic acid . The formation of the supramolecular synthon is confirmed to be the contribution of hydrogen bonding .Chemical Reactions Analysis
Dapsone exerts its anti-bacterial effects by inhibiting dihydrofolic acid synthesis, leading to inhibition of bacterial growth . It also inhibits reactive oxygen species (ROS) production, reducing the effect of eosinophil peroxidase on mast cells and downregulating neutrophil-mediated inflammatory responses .Physical And Chemical Properties Analysis
Dapsone is a white crystalline powder that is odorless. It has a purity of at least 99.5% and an apparent density at 20°C of approximately 300 Kg/m3. It has a pH of 5.5 to 7.5 when 10g/l is dissolved in water at 20°C. It is exceedingly soluble in alcohol, acetone, and MEK .Wissenschaftliche Forschungsanwendungen
Dermatological Applications : Dapsone is primarily known for its effectiveness in dermatology. It exhibits both antimicrobial/antiprotozoal effects and anti-inflammatory features. Its anti-inflammatory properties are particularly beneficial in treating chronic inflammatory disorders in dermatology (Wozel & Blasum, 2013).
Antiglioma Activity : Dapsone has shown potential in treating glioma. Research suggests that dapsone and its analogues can inhibit various neoplastic features, such as anchorage-independent growth, clonogenic survival, and directed migration in glioma cells (Karpel-Massler et al., 2017).
Impact on Male Fertility : In animal studies, dapsone administration led to significant decreases in body and reproductive organ weights, sperm parameters, and serum testosterone concentration, suggesting potential adverse effects on male fertility (Akinsomisoye, Gupta, & Raji, 2019).
Mast Cell Modulation : Dapsone has been found to inhibit mast cell activation and prevent generation and/or release of mast cell mediators, which could explain some of its therapeutic effects in disorders involving mast cells (Ruzicka, Wasserman, Soter, & Printz, 1983).
Role in Methemoglobinemia : Dapsone is a recognized cause of acquired methemoglobinemia in human patients. Its metabolite, dapsone hydroxylamine, contributes to this condition, which impairs oxygen delivery (Abouraya et al., 2012).
Neuroprotective and Cytoprotective Effects : Dapsone has been identified as a safe and effective neuro and cytoprotective agent in various models of ischemic damage, traumatic damage, and neurodegenerative diseases like Parkinson's and Alzheimer's (Diaz‐Ruiz et al., 2021).
Pharmacokinetics and Metabolism : Dapsone's pharmacokinetics and metabolism have been studied extensively, revealing insights into its acetylation and elimination processes, which are important for its clinical use (Zuidema, Hilbers-Modderman, & Merkus, 1986).
Electroanalytical Applications : An electrochemical sensor based on a molecularly imprinted polymer for dapsone determination in pharmaceutical samples has been developed, showcasing its potential in analytical chemistry (Essousi & Barhoumi, 2018).
Anticonvulsive Effects : Dapsone has displayed anticonvulsive effects in animal models of epilepsy, suggesting its potential as an antiepileptic drug (Hamada et al., 1991).
HLA-B*1301 and Dapsone Hypersensitivity
: The HLA-B*13:01 allele has been linked to the development of dapsone hypersensitivity syndrome among patients with leprosy (Zhang et al., 2013).
Hematological Effects : Dapsone's hematological side-effects, including methemoglobinemia and hemolysis, have been a focus of research. The N-hydroxy metabolites of dapsone are implicated in these effects (Jollow, Bradshaw, & McMillan, 1995).
Photodermatitis : Dapsone has been associated with photosensitivity in patients, an adverse reaction not restricted to leprosy patients (Stöckel, Meurer, & Wozel, 2001).
Topical Formulations for Acne : Dapsone's efficacy in treating acne via topical formulations has been explored, showing potential as an alternative treatment for dermatological conditions (Shamma, Salah Ad-din, & Abdeltawab, 2019).
Treatment of Pemphigus and Pemphigoid : Dapsone has shown efficacy in the treatment of pemphigus and pemphigoid, often used as a corticosteroid-sparing agent (Gürcan & Ahmed, 2009).
Granulopoiesis Inhibition : Research has shown that a metabolite of dapsone, DDS-NOH, can suppress human marrow CFUc proliferation, linking it to agranulocytosis (Weetman et al., 1980).
Toxicity and Patient Tolerance : Studies have investigated the toxicity of dapsone and strategies for increasing patient tolerance, focusing on its hemotoxic effects and potential countermeasures (Coleman, 1993).
Wirkmechanismus
Zukünftige Richtungen
Dapsone has been traditionally used as a potent anti-bacterial agent in clinical management of leprosy and has been among the first-line medications used in multidrug treatment of leprosy recommended by the World Health Organization (WHO). It has multiple antioxidant, anti-inflammatory, and anti-apoptotic functions, and it has been described in treating a wide variety of inflammatory and infectious skin conditions . Future research may explore different molecular targets for dapsone and provide further insight into the anti-inflammatory mechanism of dapsone .
Eigenschaften
IUPAC Name |
4-(4-aminophenyl)sulfonyl-2,3,5,6-tetradeuterioaniline | |
---|---|---|
Source | PubChem | |
URL | https://pubchem.ncbi.nlm.nih.gov | |
Description | Data deposited in or computed by PubChem | |
InChI |
InChI=1S/C12H12N2O2S/c13-9-1-5-11(6-2-9)17(15,16)12-7-3-10(14)4-8-12/h1-8H,13-14H2/i1D,2D,5D,6D | |
Source | PubChem | |
URL | https://pubchem.ncbi.nlm.nih.gov | |
Description | Data deposited in or computed by PubChem | |
InChI Key |
MQJKPEGWNLWLTK-NMRLXUNGSA-N | |
Source | PubChem | |
URL | https://pubchem.ncbi.nlm.nih.gov | |
Description | Data deposited in or computed by PubChem | |
Canonical SMILES |
C1=CC(=CC=C1N)S(=O)(=O)C2=CC=C(C=C2)N | |
Source | PubChem | |
URL | https://pubchem.ncbi.nlm.nih.gov | |
Description | Data deposited in or computed by PubChem | |
Isomeric SMILES |
[2H]C1=C(C(=C(C(=C1N)[2H])[2H])S(=O)(=O)C2=CC=C(C=C2)N)[2H] | |
Source | PubChem | |
URL | https://pubchem.ncbi.nlm.nih.gov | |
Description | Data deposited in or computed by PubChem | |
Molecular Formula |
C12H12N2O2S | |
Source | PubChem | |
URL | https://pubchem.ncbi.nlm.nih.gov | |
Description | Data deposited in or computed by PubChem | |
Molecular Weight |
252.33 g/mol | |
Source | PubChem | |
URL | https://pubchem.ncbi.nlm.nih.gov | |
Description | Data deposited in or computed by PubChem | |
Synthesis routes and methods I
Procedure details
Synthesis routes and methods II
Procedure details
Synthesis routes and methods III
Procedure details
Q & A
Q1: How does the choice of Dapsone-d4 as an internal standard impact the accuracy of Dapsone quantification in plasma samples using LC-ESI-MS?
A2: The research article demonstrates that despite the observed signal suppression of Dapsone-d4 by Dapsone, the use of Dapsone-d4 as an internal standard still allows for accurate Dapsone quantification in plasma samples. [] The researchers observed that the slopes of the calibration curves for Dapsone, using Dapsone-d4 as the internal standard, remained close to unity (0.905 +/- 0.048). This suggests that the signal suppression affects both the analyte and the internal standard proportionally, allowing the isotope dilution method to effectively correct for the suppression effect. []
Haftungsausschluss und Informationen zu In-Vitro-Forschungsprodukten
Bitte beachten Sie, dass alle Artikel und Produktinformationen, die auf BenchChem präsentiert werden, ausschließlich zu Informationszwecken bestimmt sind. Die auf BenchChem zum Kauf angebotenen Produkte sind speziell für In-vitro-Studien konzipiert, die außerhalb lebender Organismen durchgeführt werden. In-vitro-Studien, abgeleitet von dem lateinischen Begriff "in Glas", beinhalten Experimente, die in kontrollierten Laborumgebungen unter Verwendung von Zellen oder Geweben durchgeführt werden. Es ist wichtig zu beachten, dass diese Produkte nicht als Arzneimittel oder Medikamente eingestuft sind und keine Zulassung der FDA für die Vorbeugung, Behandlung oder Heilung von medizinischen Zuständen, Beschwerden oder Krankheiten erhalten haben. Wir müssen betonen, dass jede Form der körperlichen Einführung dieser Produkte in Menschen oder Tiere gesetzlich strikt untersagt ist. Es ist unerlässlich, sich an diese Richtlinien zu halten, um die Einhaltung rechtlicher und ethischer Standards in Forschung und Experiment zu gewährleisten.