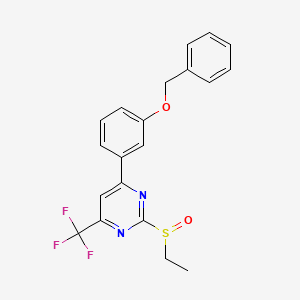
Betp
Übersicht
Beschreibung
Wirkmechanismus
Target of Action
BetP, also known as the betaine symporter, is a protein that plays a crucial role in the osmotic stress response in certain bacteria, such as Corynebacterium glutamicum . Its primary targets are the glucocorticoid receptors . These receptors are part of the larger family of steroid hormone receptors and play a significant role in regulating various physiological processes, including immune response, metabolism, and cell growth .
Mode of Action
This compound is a prodrug that is rapidly hydrolyzed, providing rapidly accessible betamethasone to agonize glucocorticoid receptors . This interaction with its targets leads to greater anti-inflammatory activity than prednisolone, with less sodium and water retention .
Biochemical Pathways
This compound affects several biochemical pathways. It senses changes in the membrane state and increasing levels of cytoplasmic K during hyperosmotic stress via its C-terminal domain and regulates transport activity according to both stimuli . Activation and regulation depend crucially on the lipid composition of the surrounding membrane . This compound is active and regulated only when negatively charged lipids are present .
Pharmacokinetics
The pharmacokinetics of this compound were examined in rats after intravenous and oral dosing .
Result of Action
The molecular and cellular effects of this compound’s action are primarily related to its anti-inflammatory activity. By agonizing glucocorticoid receptors, this compound provides greater anti-inflammatory activity than prednisolone . This can lead to a reduction in inflammation and associated symptoms in various inflammatory conditions.
Action Environment
The action, efficacy, and stability of this compound can be influenced by various environmental factors. For instance, the lipid composition of the surrounding membrane plays a crucial role in the activation and regulation of this compound . Specifically, this compound is active and regulated only when negatively charged lipids are present . This suggests that changes in the lipid environment could potentially influence the action of this compound.
Biochemische Analyse
Biochemical Properties
BetP transports betaine with high specificity, which under hyperosmotic conditions is accumulated in molar amounts against the concentration gradient into the cytoplasm . The transporter exploits the inward directed sodium gradient across the membrane to energize betaine transport in a stoichiometry of 2 Na+:1 betaine . It is assumed that this compound senses changes of the physical state of the membrane via its C-terminal domain .
Cellular Effects
This compound has a significant impact on cellular processes, particularly in response to osmotic stress. It senses an osmotic upshift via its osmosensory C-terminal domain and responds rapidly with a steep increase in transport rate . This rapid response helps the cell to adapt to changes in the external environment and maintain homeostasis.
Molecular Mechanism
The molecular mechanism of this compound involves a complex interplay of structural changes and interactions with other molecules. X-ray crystallography has identified four putative K+ interaction sites at the C-terminal domains of this compound . The presence of K+ results in asymmetric partial unfolding of the C-terminal domains, which is an intermediate between the downregulated state and the conformation observed in crystal structures .
Temporal Effects in Laboratory Settings
In laboratory settings, the effects of this compound can change over time. For instance, the activation of this compound is highly cooperative, with K+ binding to this compound in vivo showing a high degree of cooperativity . This suggests that the activity of this compound can be modulated by changes in the intracellular environment.
Dosage Effects in Animal Models
While specific dosage effects of this compound in animal models have not been extensively studied, it is known that the activity of this compound is regulated by the intracellular concentration of K+ . This suggests that the effects of this compound could potentially vary with different dosages of K+.
Metabolic Pathways
This compound is involved in the transport of betaine, a process that is crucial for the osmotic stress response in bacteria . This process is part of a larger metabolic pathway that helps the cell to adapt to changes in the external environment.
Transport and Distribution
This compound is a membrane transporter, and it is distributed within the cell membrane . It is thought to sense changes in the physical state of the membrane via its C-terminal domain , which may affect its distribution within the membrane.
Subcellular Localization
This compound is localized in the cell membrane, where it functions as a transporter . The C-terminal domain of this compound, which is thought to be involved in sensing osmotic stress, is attached at the membrane surface when this compound is downregulated in the absence of stress .
Vorbereitungsmethoden
Synthesewege und Reaktionsbedingungen
BETP kann durch einen mehrstufigen Prozess synthetisiert werden, der die Bildung des Pyrimidinkernes beinhaltet, gefolgt von der Einführung der Benzyloxyphenyl-, Ethylsulfinyl- und Trifluormethylgruppen. Die Synthese umfasst typischerweise:
Bildung des Pyrimidinkernes: Dies kann durch die Reaktion geeigneter Vorläufer unter Bedingungen erreicht werden, die die Cyclisierung fördern.
Einführung der Benzyloxyphenylgruppe: Dieser Schritt beinhaltet die Kupplung eines Benzyloxyphenylderivats mit dem Pyrimidinkern unter Verwendung einer palladiumkatalysierten Kreuzkupplungsreaktion.
Einführung der Ethylsulfinylgruppe: Dies kann durch die Oxidation einer Ethylthiogruppe zu einer Ethylsulfinylgruppe unter Verwendung eines Oxidationsmittels wie Wasserstoffperoxid erfolgen.
Einführung der Trifluormethylgruppe: Dieser Schritt beinhaltet die Addition einer Trifluormethylgruppe an den Pyrimidinkern unter Verwendung eines Reagenzes wie Trifluormethyliodid.
Industrielle Produktionsmethoden
Die industrielle Produktion von this compound folgt ähnlichen Synthesewegen, wird jedoch für die großtechnische Produktion optimiert. Dies beinhaltet die Verwendung von Hochdurchsatzscreening und Optimierung der Reaktionsbedingungen, um Ausbeute und Reinheit zu maximieren. Das Verfahren kann auch die Verwendung von automatisierten Syntheseanlagen und kontinuierlichen Durchflussreaktoren umfassen, um Effizienz und Skalierbarkeit zu verbessern .
Analyse Chemischer Reaktionen
Arten von Reaktionen
BETP unterliegt verschiedenen chemischen Reaktionen, darunter:
Oxidation: Die Ethylthiogruppe kann zu einer Ethylsulfinylgruppe oxidiert werden.
Reduktion: Die Ethylsulfinylgruppe kann zurück zu einer Ethylthiogruppe reduziert werden.
Substitution: Die Trifluormethylgruppe kann unter geeigneten Bedingungen durch andere funktionelle Gruppen substituiert werden.
Häufige Reagenzien und Bedingungen
Oxidation: Wasserstoffperoxid wird häufig als Oxidationsmittel verwendet.
Reduktion: Reduktionsmittel wie Natriumborhydrid können verwendet werden.
Substitution: Verschiedene Nucleophile können für Substitutionsreaktionen verwendet werden, abhängig von der gewünschten funktionellen Gruppe.
Hauptprodukte
Die Hauptprodukte, die aus diesen Reaktionen gebildet werden, umfassen Derivate von this compound mit modifizierten funktionellen Gruppen, wie z. B. Ethylthio-, Ethylsulfinyl- und substituierte Trifluormethylgruppen .
Wissenschaftliche Forschungsanwendungen
BETP hat mehrere Anwendungen in der wissenschaftlichen Forschung, darunter:
Chemie: this compound wird als Werkzeugverbindung verwendet, um den Glucagon-like Peptide-1-Rezeptor und seine Rolle im Glukosestoffwechsel zu untersuchen.
Biologie: this compound wird verwendet, um die Signalwege zu untersuchen, die an der Insulinsekretion und der Glukosehomöostase beteiligt sind.
Medizin: this compound wird als potenzieller Therapeutika für die Behandlung von Typ-2-Diabetes mellitus und Fettleibigkeit untersucht.
Wirkmechanismus
This compound entfaltet seine Wirkung durch Bindung an den Glucagon-like Peptide-1-Rezeptor, einen G-Protein-gekoppelten Rezeptor. Nach der Bindung wirkt this compound als positiver allosterischer Modulator und verstärkt die Reaktion des Rezeptors auf seinen natürlichen Liganden, Glucagon-like Peptide-1. Dies führt zu einer erhöhten Insulinsekretion und einem verbesserten Glukosestoffwechsel. Die beteiligten molekularen Ziele umfassen den Rezeptor selbst und nachgeschaltete Signalwege wie den zyklischen Adenosinmonophosphat-Weg .
Vergleich Mit ähnlichen Verbindungen
BETP ist unter den Agonisten des Glucagon-like Peptide-1-Rezeptors einzigartig aufgrund seiner kleinen Molekülgröße und seiner oralen Bioverfügbarkeit. Ähnliche Verbindungen umfassen:
Liraglutid: Ein Peptidagonist des Glucagon-like Peptide-1-Rezeptors, der per Injektion verabreicht wird.
Semaglutid: Ein weiterer Peptidagonist, der ebenfalls per Injektion verabreicht wird.
Danuglipron: Ein kleiner Molekülagonist ähnlich this compound, aber mit unterschiedlichen pharmakokinetischen Eigenschaften.
Die Einzigartigkeit von this compound liegt in seiner Fähigkeit, oral verabreicht zu werden, was die Compliance des Patienten im Vergleich zu injizierbaren Peptidagonisten verbessert .
Eigenschaften
IUPAC Name |
2-ethylsulfinyl-4-(3-phenylmethoxyphenyl)-6-(trifluoromethyl)pyrimidine | |
---|---|---|
Source | PubChem | |
URL | https://pubchem.ncbi.nlm.nih.gov | |
Description | Data deposited in or computed by PubChem | |
InChI |
InChI=1S/C20H17F3N2O2S/c1-2-28(26)19-24-17(12-18(25-19)20(21,22)23)15-9-6-10-16(11-15)27-13-14-7-4-3-5-8-14/h3-12H,2,13H2,1H3 | |
Source | PubChem | |
URL | https://pubchem.ncbi.nlm.nih.gov | |
Description | Data deposited in or computed by PubChem | |
InChI Key |
NTDFYGSSDDMNHI-UHFFFAOYSA-N | |
Source | PubChem | |
URL | https://pubchem.ncbi.nlm.nih.gov | |
Description | Data deposited in or computed by PubChem | |
Canonical SMILES |
CCS(=O)C1=NC(=CC(=N1)C(F)(F)F)C2=CC(=CC=C2)OCC3=CC=CC=C3 | |
Source | PubChem | |
URL | https://pubchem.ncbi.nlm.nih.gov | |
Description | Data deposited in or computed by PubChem | |
Molecular Formula |
C20H17F3N2O2S | |
Source | PubChem | |
URL | https://pubchem.ncbi.nlm.nih.gov | |
Description | Data deposited in or computed by PubChem | |
Molecular Weight |
406.4 g/mol | |
Source | PubChem | |
URL | https://pubchem.ncbi.nlm.nih.gov | |
Description | Data deposited in or computed by PubChem | |
CAS No. |
1371569-69-5 | |
Record name | 1371569-69-5 | |
Source | European Chemicals Agency (ECHA) | |
URL | https://echa.europa.eu/information-on-chemicals | |
Description | The European Chemicals Agency (ECHA) is an agency of the European Union which is the driving force among regulatory authorities in implementing the EU's groundbreaking chemicals legislation for the benefit of human health and the environment as well as for innovation and competitiveness. | |
Explanation | Use of the information, documents and data from the ECHA website is subject to the terms and conditions of this Legal Notice, and subject to other binding limitations provided for under applicable law, the information, documents and data made available on the ECHA website may be reproduced, distributed and/or used, totally or in part, for non-commercial purposes provided that ECHA is acknowledged as the source: "Source: European Chemicals Agency, http://echa.europa.eu/". Such acknowledgement must be included in each copy of the material. ECHA permits and encourages organisations and individuals to create links to the ECHA website under the following cumulative conditions: Links can only be made to webpages that provide a link to the Legal Notice page. | |
Q1: What is the primary function of BetP?
A1: this compound is a secondary active transporter that facilitates the uptake of the compatible solute glycine betaine into the cytoplasm of Corynebacterium glutamicum [, ]. This process is driven by the co-transport of sodium ions [, ].
Q2: What is the physiological role of glycine betaine accumulation in C. glutamicum?
A2: Glycine betaine acts as a compatible solute, protecting the cell from the detrimental effects of hyperosmotic stress [, ]. It helps maintain cellular turgor pressure and enzyme function under high osmolarity conditions [, , ].
Q3: How does this compound contribute to the osmotic stress response of C. glutamicum?
A3: this compound acts as both an osmosensor and an osmoregulator [, , , ]. It senses hyperosmotic conditions and responds by increasing its transport rate to rapidly accumulate glycine betaine [, ].
Q4: Does C. glutamicum rely solely on this compound for compatible solute uptake?
A4: No, C. glutamicum possesses four secondary carriers for compatible solutes: this compound, EctP, LcoP, and ProP, each with distinct substrate specificities and affinities [, ].
Q5: What is the oligomeric state of this compound?
A5: this compound functions as a homotrimer, meaning it is composed of three identical protein subunits [, , ].
Q6: Which domains of this compound are involved in sensing osmotic stress?
A6: Both the N-terminal and C-terminal cytoplasmic extensions of this compound contribute to osmosensing and regulation [, ].
Q7: What is the role of the C-terminal domain in this compound regulation?
A7: The C-terminal domain acts as a primary osmosensor, directly sensing changes in the cytoplasmic potassium ion (K+) concentration as an indicator of hyperosmotic stress [, , ].
Q8: How does the C-terminal domain transduce the osmotic signal?
A8: Upon K+ binding, the C-terminal domain undergoes conformational changes, leading to a rearrangement of the this compound trimer and activation of the transporter [, ]. This involves switching interactions between the C-terminal domain, the N-terminal domain, and the membrane lipids [, ].
Q9: What is the role of the N-terminal domain in this compound regulation?
A9: The N-terminal domain contains negatively charged clusters that interact with the positively charged C-terminal domain, modulating the activation state of this compound []. This interaction is crucial for the osmoregulatory response.
Q10: What is the significance of the five transmembrane-helix inverted-topology repeat (FIRL) fold in this compound?
A10: this compound, like other sodium-coupled transporters, adopts the FIRL fold, which is believed to be essential for the alternating access mechanism that allows for substrate transport across the membrane [].
Q11: What are the primary stimuli that activate this compound?
A11: this compound is activated by: * Increased internal K+ concentration: This is the primary signal for hyperosmotic stress response [, ].* Changes in the physical state of the membrane: The exact nature of this signal is not fully elucidated, but it is thought to involve alterations in membrane fluidity or lipid composition [, , ].
Q12: How does K+ activate this compound?
A12: K+ binds to specific sites within the C-terminal domain of this compound []. This binding is highly cooperative and triggers conformational changes that ultimately lead to transporter activation [, ].
Q13: How do changes in membrane composition affect this compound activity?
A13: The presence of negatively charged lipids, particularly phosphatidylglycerol, is crucial for this compound activity and osmoregulation [, ]. The C-terminal domain interacts with these lipids, and these interactions are modulated by K+ binding [].
Q14: Is this compound subject to regulation after initial activation?
A14: Yes, during the adaptation phase to hyperosmotic stress, this compound activity decreases despite the continued presence of a high internal K+ concentration []. This adaptation regulation is independent of K+ and might be related to changes in the hydration status of this compound or altered protein-membrane interactions [].
Q15: Does this compound exhibit any transport activity in the absence of osmotic stress?
A15: Yes, this compound displays a basal level of activity even under low osmolarity conditions. This ensures a minimal uptake of compatible solutes and allows for a rapid response upon osmotic upshift [].
Q16: What structural techniques have been employed to study this compound?
A16: Several techniques have been used to elucidate this compound structure, including:* X-ray crystallography: Provided high-resolution structures of this compound in various conformations [, ].* Cryo-electron microscopy (CryoEM): Revealed the structure of this compound in a lipid bilayer environment [].* Electron paramagnetic resonance (EPR) spectroscopy: Provided insights into the dynamics of this compound in response to different stimuli [, ].* Single-molecule FRET: Offered information about conformational changes and heterogeneity of this compound [].
Q17: What insights have structural studies provided into the mechanism of this compound activation?
A17: Structural studies, combined with biochemical and biophysical data, suggest that this compound undergoes a series of conformational changes during activation [, ]. * In the absence of K+, the C-terminal domains are located near the membrane surface, representing a downregulated state []. * Upon K+ binding, the C-terminal domains partially unfold and reorient, leading to a rearrangement of the transporter and facilitating substrate binding and transport [, ].
Q18: How does this compound compare to other osmoregulated transporters?
A18: While this compound's specific K+-dependent regulation is unique, the concept of sensing domain unfolding/refolding as a mechanism for responding to hyperosmotic stress is likely shared by other osmoregulated transporters [].
Q19: Are there any biotechnological applications of this compound or related transporters?
A19: Understanding the mechanisms of osmoregulation in bacteria like C. glutamicum has implications for optimizing their use in biotechnology [, ]. Engineering strains with enhanced osmotolerance could improve their performance in industrial processes.
Haftungsausschluss und Informationen zu In-Vitro-Forschungsprodukten
Bitte beachten Sie, dass alle Artikel und Produktinformationen, die auf BenchChem präsentiert werden, ausschließlich zu Informationszwecken bestimmt sind. Die auf BenchChem zum Kauf angebotenen Produkte sind speziell für In-vitro-Studien konzipiert, die außerhalb lebender Organismen durchgeführt werden. In-vitro-Studien, abgeleitet von dem lateinischen Begriff "in Glas", beinhalten Experimente, die in kontrollierten Laborumgebungen unter Verwendung von Zellen oder Geweben durchgeführt werden. Es ist wichtig zu beachten, dass diese Produkte nicht als Arzneimittel oder Medikamente eingestuft sind und keine Zulassung der FDA für die Vorbeugung, Behandlung oder Heilung von medizinischen Zuständen, Beschwerden oder Krankheiten erhalten haben. Wir müssen betonen, dass jede Form der körperlichen Einführung dieser Produkte in Menschen oder Tiere gesetzlich strikt untersagt ist. Es ist unerlässlich, sich an diese Richtlinien zu halten, um die Einhaltung rechtlicher und ethischer Standards in Forschung und Experiment zu gewährleisten.