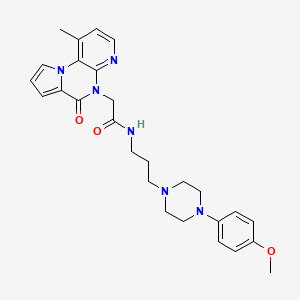
ML 190
Übersicht
Beschreibung
ML190 ist ein selektiver κ-Opioid-Rezeptor-Antagonist. Es ist bekannt für seine hohe Affinität und Selektivität gegenüber dem κ-Opioid-Rezeptor mit einem IC50-Wert von 120 nM und einem EC50-Wert von 129 nM . Diese Verbindung wurde umfassend auf ihre potenziellen therapeutischen Anwendungen, insbesondere im Bereich der Neurowissenschaften, untersucht.
Herstellungsmethoden
Synthesewege und Reaktionsbedingungen
Die Synthese von ML190 umfasst mehrere Schritte, beginnend mit kommerziell erhältlichen Ausgangsmaterialien. Das Endprodukt wird durch eine Reihe von Reinigungsschritten erhalten, darunter Umkristallisation und Chromatographie .
Industrielle Produktionsmethoden
Die industrielle Produktion von ML190 folgt ähnlichen Synthesewegen, jedoch in größerem Maßstab. Der Prozess umfasst die Optimierung der Reaktionsbedingungen, um eine hohe Ausbeute und Reinheit zu gewährleisten. Die Verwendung von automatisierten Reaktoren und kontinuierlichen Fließsystemen kann die Effizienz und Skalierbarkeit des Produktionsprozesses verbessern .
Vorbereitungsmethoden
Synthetic Routes and Reaction Conditions
The synthesis of ML190 involves multiple steps, starting from commercially available starting materialsThe final product is obtained through a series of purification steps, including recrystallization and chromatography .
Industrial Production Methods
Industrial production of ML190 follows similar synthetic routes but on a larger scale. The process involves optimization of reaction conditions to ensure high yield and purity. The use of automated reactors and continuous flow systems can enhance the efficiency and scalability of the production process .
Analyse Chemischer Reaktionen
Arten von Reaktionen
ML190 unterliegt verschiedenen chemischen Reaktionen, darunter:
Oxidation: ML190 kann unter bestimmten Bedingungen oxidiert werden, um entsprechende oxidierte Produkte zu bilden.
Reduktion: Reduktionsreaktionen können durchgeführt werden, um die in ML190 vorhandenen funktionellen Gruppen zu modifizieren.
Häufige Reagenzien und Bedingungen
Oxidation: Häufige Oxidationsmittel wie Kaliumpermanganat oder Chromtrioxid können verwendet werden.
Reduktion: Reduktionsmittel wie Natriumborhydrid oder Lithiumaluminiumhydrid werden häufig eingesetzt.
Substitution: Verschiedene Nucleophile und Elektrophile können unter geeigneten Bedingungen verwendet werden, um Substitutionsreaktionen zu erzielen.
Hauptprodukte, die gebildet werden
Die Hauptprodukte, die gebildet werden
Eigenschaften
IUPAC Name |
N-[3-[4-(4-methoxyphenyl)piperazin-1-yl]propyl]-2-(13-methyl-7-oxo-2,8,10-triazatricyclo[7.4.0.02,6]trideca-1(13),3,5,9,11-pentaen-8-yl)acetamide | |
---|---|---|
Source | PubChem | |
URL | https://pubchem.ncbi.nlm.nih.gov | |
Description | Data deposited in or computed by PubChem | |
InChI |
InChI=1S/C27H32N6O3/c1-20-10-12-29-26-25(20)32-14-3-5-23(32)27(35)33(26)19-24(34)28-11-4-13-30-15-17-31(18-16-30)21-6-8-22(36-2)9-7-21/h3,5-10,12,14H,4,11,13,15-19H2,1-2H3,(H,28,34) | |
Source | PubChem | |
URL | https://pubchem.ncbi.nlm.nih.gov | |
Description | Data deposited in or computed by PubChem | |
InChI Key |
PMTIWRPLQBVEMR-UHFFFAOYSA-N | |
Source | PubChem | |
URL | https://pubchem.ncbi.nlm.nih.gov | |
Description | Data deposited in or computed by PubChem | |
Canonical SMILES |
CC1=C2C(=NC=C1)N(C(=O)C3=CC=CN32)CC(=O)NCCCN4CCN(CC4)C5=CC=C(C=C5)OC | |
Source | PubChem | |
URL | https://pubchem.ncbi.nlm.nih.gov | |
Description | Data deposited in or computed by PubChem | |
Molecular Formula |
C27H32N6O3 | |
Source | PubChem | |
URL | https://pubchem.ncbi.nlm.nih.gov | |
Description | Data deposited in or computed by PubChem | |
Molecular Weight |
488.6 g/mol | |
Source | PubChem | |
URL | https://pubchem.ncbi.nlm.nih.gov | |
Description | Data deposited in or computed by PubChem | |
Synthesis routes and methods
Procedure details
Haftungsausschluss und Informationen zu In-Vitro-Forschungsprodukten
Bitte beachten Sie, dass alle Artikel und Produktinformationen, die auf BenchChem präsentiert werden, ausschließlich zu Informationszwecken bestimmt sind. Die auf BenchChem zum Kauf angebotenen Produkte sind speziell für In-vitro-Studien konzipiert, die außerhalb lebender Organismen durchgeführt werden. In-vitro-Studien, abgeleitet von dem lateinischen Begriff "in Glas", beinhalten Experimente, die in kontrollierten Laborumgebungen unter Verwendung von Zellen oder Geweben durchgeführt werden. Es ist wichtig zu beachten, dass diese Produkte nicht als Arzneimittel oder Medikamente eingestuft sind und keine Zulassung der FDA für die Vorbeugung, Behandlung oder Heilung von medizinischen Zuständen, Beschwerden oder Krankheiten erhalten haben. Wir müssen betonen, dass jede Form der körperlichen Einführung dieser Produkte in Menschen oder Tiere gesetzlich strikt untersagt ist. Es ist unerlässlich, sich an diese Richtlinien zu halten, um die Einhaltung rechtlicher und ethischer Standards in Forschung und Experiment zu gewährleisten.