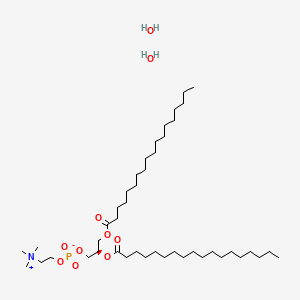
1,2-Distearoyl-sn-glycero-3-phosphocholine dihydrate
Übersicht
Beschreibung
1,2-Distearoyl-<i>sn</i>-glycero-3-phosphocholine (DSPC) dihydrate is a saturated phospholipid with two stearic acid (18:0) acyl chains esterified to the glycerol backbone and a zwitterionic phosphocholine head group. Its fully saturated structure confers high phase transition temperature (Tm ~55°C), enabling stable lipid bilayer formation at physiological temperatures . DSPC is a critical component of lipid nanoparticles (LNPs) in mRNA vaccines (e.g., Pfizer-BioNTech’s BNT162b2 and Moderna’s mRNA-1273), where it stabilizes the lipid bilayer and enhances nanoparticle integrity during storage and delivery .
Vorbereitungsmethoden
Synthetic Routes and Reaction Conditions
1,2-Distearoyl-sn-glycero-3-phosphocholine dihydrate can be synthesized through the esterification of glycerol with stearic acid, followed by phosphorylation. The reaction typically involves the use of catalysts and specific reaction conditions to ensure high yield and purity .
Industrial Production Methods
Industrial production of this compound involves large-scale esterification and phosphorylation processes. These methods are optimized for efficiency and cost-effectiveness, often utilizing continuous flow reactors and advanced purification techniques .
Analyse Chemischer Reaktionen
Types of Reactions
1,2-Distearoyl-sn-glycero-3-phosphocholine dihydrate undergoes various chemical reactions, including:
Oxidation: This reaction can lead to the formation of peroxides and other oxidative products.
Reduction: Reduction reactions can modify the phospholipid head group or the fatty acid chains.
Substitution: Substitution reactions can occur at the phosphate group or the glycerol backbone.
Common Reagents and Conditions
Common reagents used in these reactions include oxidizing agents like hydrogen peroxide, reducing agents such as sodium borohydride, and various catalysts to facilitate substitution reactions. The conditions often involve controlled temperatures and pH levels to ensure specific reaction pathways .
Major Products
The major products formed from these reactions depend on the specific reagents and conditions used. For example, oxidation can produce hydroperoxides, while reduction can yield modified phospholipids with altered head groups or fatty acid chains .
Wissenschaftliche Forschungsanwendungen
1,2-Distearoyl-sn-glycero-3-phosphocholine dihydrate has numerous applications in scientific research:
Wirkmechanismus
The mechanism of action of 1,2-Distearoyl-sn-glycero-3-phosphocholine dihydrate involves its incorporation into lipid bilayers, where it influences membrane fluidity and stability. It interacts with other lipids and proteins, affecting various cellular processes. The molecular targets include cell membrane components and signaling pathways involved in lipid metabolism and transport .
Vergleich Mit ähnlichen Verbindungen
Comparative Analysis with Structurally Similar Phospholipids
Phosphatidylcholines (PCs) vary in acyl chain length, saturation, and head-group modifications, which directly influence their physicochemical properties and applications. Below is a detailed comparison of DSPC with analogous compounds:
Acyl Chain Length and Saturation
Table 1: Structural and Functional Comparison of Key Phosphatidylcholines
Key Findings:
- Chain Length and Tm : Longer saturated acyl chains (e.g., DSPC’s 18:0 vs. DMPC’s 14:0) increase hydrophobic interactions, raising Tm and enhancing thermal stability . DSPC’s high Tm ensures LNP integrity during refrigeration (2–8°C), critical for mRNA vaccine storage .
- Saturation and Membrane Fluidity : Unsaturated PCs like DOPC (18:1) have low Tm due to kinked acyl chains, enabling membrane fusion and endosomal escape in LNPs . However, DSPC’s rigidity prevents premature mRNA release, balancing stability and controlled delivery .
Head-Group Modifications
DSPC’s phosphocholine head group is neutral at physiological pH, reducing nonspecific interactions with serum proteins and improving circulation time . In contrast, charged phospholipids like 1,2-distearoyl-<i>sn</i>-glycero-3-phospho-(1'-rac-glycerol) (DSPG) introduce negative charges, promoting immune cell uptake but increasing clearance rates .
Role in Drug Delivery Systems
- DSPC vs. Cholesterol : In LNPs, DSPC complements cholesterol (rigidity enhancer) by forming a tightly packed bilayer, while cholesterol prevents crystallization and improves encapsulation efficiency .
- DSPC vs. PEGylated Lipids : PEGylated lipids (e.g., ALC-0159) shield LNPs from immune recognition, whereas DSPC provides structural cohesion .
Biologische Aktivität
1,2-Distearoyl-sn-glycero-3-phosphocholine (DSPC) is a phospholipid that plays a crucial role in various biological applications, particularly in the formulation of lipid nanoparticles (LNPs) for drug delivery. This article explores the biological activity of DSPC, focusing on its physicochemical properties, applications in drug delivery systems, and relevant case studies.
DSPC is characterized by the following chemical properties:
Property | Value |
---|---|
Molecular Formula | C₄₄H₈₈NO₈P |
Molecular Weight | 790.145 g/mol |
Melting Point | 236 °C |
CAS Number | 816-94-4 |
DSPC is a cylindrical-shaped lipid that forms stable bilayers, making it suitable for encapsulating hydrophilic and hydrophobic compounds. Its high melting point contributes to its stability in various formulations .
1. Lipid Nanoparticle Formulation
DSPC is extensively used in the formulation of lipid nanoparticles, which are critical for delivering therapeutic agents such as mRNA vaccines. For instance, during the COVID-19 pandemic, DSPC was a key component in mRNA-LNP formulations that enabled effective delivery of the vaccine components to target cells. The lipid nanoparticles protect mRNA from degradation and facilitate its uptake by cells, leading to protein expression necessary for immune response .
2. Selective Organ Targeting
Recent studies have demonstrated that DSPC can be utilized in selective organ-targeting (SORT) lipid nanoparticles. By modifying the lipid composition, researchers have been able to direct the delivery of therapeutic agents to specific organs such as the liver and lungs. For example, in formulations combining DSPC with other lipids, specific targeting was achieved through varying the molar ratios of cationic and anionic lipids .
Study 1: Toxicological Evaluation
A study evaluated the safety profile of DSPC when used in inhalation formulations. The findings indicated that DSPC and similar phospholipids are generally recognized as safe (GRAS) materials, being endogenous to lung tissue. This study highlights the potential for using DSPC in pulmonary drug delivery systems .
Study 2: Stability and Efficacy of LNPs
In a comparative analysis of different lipid formulations for drug delivery, DSPC-based LNPs exhibited superior stability and efficacy in delivering bioactive compounds compared to other lipids. The study showed that LNPs containing DSPC maintained their structural integrity over time and demonstrated enhanced cellular uptake in vitro .
The biological activity of DSPC can be attributed to its ability to form stable lipid bilayers that mimic cellular membranes. This property facilitates:
- Encapsulation Efficiency: High encapsulation rates for both hydrophilic and hydrophobic drugs.
- Cell Membrane Fusion: Enhanced interaction with cell membranes due to its structural compatibility.
- Controlled Release: The ability to modulate release kinetics based on environmental conditions such as pH and temperature.
Q & A
Basic Research Questions
Q. What are the established methods for synthesizing and characterizing DSPC dihydrate in academic research?
DSPC is synthesized via esterification of sn-glycero-3-phosphocholine with stearic acid derivatives under anhydrous conditions. Purification involves column chromatography or recrystallization. Characterization uses HPLC with evaporative light scattering detection (ELSD) for purity (≥99%) and GC with polar columns (e.g., Agilent CP-Wax 52 CB) to confirm fatty acid composition .
Q. How should DSPC dihydrate be stored to maintain stability in experimental settings?
DSPC must be stored at −20°C in airtight, light-protected containers to prevent hydrolysis. Stability is enhanced by avoiding oxidants and humidity. Pre-use validation via thin-layer chromatography (TLC) or NMR ensures lipid integrity after prolonged storage .
Q. What protocols are recommended for preparing DSPC-based liposomes for drug delivery studies?
Liposomes are typically prepared using thin-film hydration: DSPC is dissolved in chloroform, dried under vacuum, hydrated with buffer (e.g., PBS), and extruded through polycarbonate membranes. Size uniformity is confirmed via dynamic light scattering (DLS), and encapsulation efficiency is quantified using fluorescence assays or HPLC .
Advanced Research Questions
Q. How can researchers optimize DSPC-based lipid nanoparticles (LNPs) for mRNA encapsulation, and what factors contribute to batch variability?
LNPs are formulated via microfluidic mixing of DSPC, cholesterol, ionizable lipids, and mRNA. Critical parameters include flow rate ratios (aqueous:organic phases), lipid:mRNA mass ratios, and buffer pH. Variability is minimized by standardizing lipid sourcing (≥99% purity) and using orthogonal analytics (e.g., cryo-TEM for morphology, Ribogreen assays for encapsulation) .
Q. What experimental strategies resolve contradictions in reported phase transition temperatures (TmT_mTm) of DSPC membranes across studies?
Discrepancies in (typically ~55°C) arise from differences in hydration levels, buffer ionic strength, and DSC measurement protocols. Researchers should pre-equilibrate samples at controlled humidity and use high-purity DSPC. Validation via differential scanning calorimetry (DSC) with standardized heating rates (e.g., 1°C/min) improves reproducibility .
Q. How do pH-responsive DSPC formulations achieve charge conversion in targeted drug delivery, and what analytics validate this behavior?
DSPC is functionalized with pH-sensitive polymers (e.g., poly(β-amino esters)) via microfluidic coating. Charge conversion from neutral to cationic at tumor pH (6.5–6.8) is confirmed via ζ-potential titration. Cellular uptake efficiency is assessed using confocal microscopy with fluorescently tagged nanoparticles .
Q. What methodologies address low encapsulation efficiency of hydrophilic drugs in DSPC liposomes?
Active loading techniques (e.g., pH gradient or ammonium sulfate methods) improve encapsulation. Comparative studies using passive vs. active loading show that optimizing internal buffer pH (e.g., citrate pH 4.0) and drug-lipid ratios (1:10–1:20 w/w) enhances efficiency. Post-encapsulation purification via size-exclusion chromatography removes unencapsulated drug .
Q. Methodological and Analytical Considerations
Q. How are USP pharmacopeial standards applied to validate DSPC dihydrate in regulatory-compliant research?
USP monographs require GC analysis of fatty acid composition (≥99% stearic acid) and HPLC-ELSD for phospholipid purity. Positional specificity of acyl chains is confirmed via enzymatic digestion with phospholipase A2, followed by LC-MS to detect lyso-phosphatidylcholine products .
Q. What advanced imaging techniques characterize DSPC membrane interactions with proteins or small molecules?
Neutron scattering and atomic force microscopy (AFM) reveal lipid bilayer organization and protein insertion dynamics. Förster resonance energy transfer (FRET) assays quantify molecular interactions using fluorescently labeled DSPC and ligands .
Q. How do researchers mitigate oxidative degradation of DSPC during long-term in vitro studies?
Antioxidants (e.g., α-tocopherol, 0.1–1 mol%) are incorporated into lipid formulations. Oxidative byproducts are monitored via malondialdehyde (MDA) assays or LC-MS for truncated lipid species. Storage under inert gas (N₂/Ar) further prevents degradation .
Eigenschaften
IUPAC Name |
[(2R)-2,3-di(octadecanoyloxy)propyl] 2-(trimethylazaniumyl)ethyl phosphate;dihydrate | |
---|---|---|
Source | PubChem | |
URL | https://pubchem.ncbi.nlm.nih.gov | |
Description | Data deposited in or computed by PubChem | |
InChI |
InChI=1S/C44H88NO8P.2H2O/c1-6-8-10-12-14-16-18-20-22-24-26-28-30-32-34-36-43(46)50-40-42(41-52-54(48,49)51-39-38-45(3,4)5)53-44(47)37-35-33-31-29-27-25-23-21-19-17-15-13-11-9-7-2;;/h42H,6-41H2,1-5H3;2*1H2/t42-;;/m1../s1 | |
Source | PubChem | |
URL | https://pubchem.ncbi.nlm.nih.gov | |
Description | Data deposited in or computed by PubChem | |
InChI Key |
VHRFFOPIZMXKSG-YHZVPMRTSA-N | |
Source | PubChem | |
URL | https://pubchem.ncbi.nlm.nih.gov | |
Description | Data deposited in or computed by PubChem | |
Canonical SMILES |
CCCCCCCCCCCCCCCCCC(=O)OCC(COP(=O)([O-])OCC[N+](C)(C)C)OC(=O)CCCCCCCCCCCCCCCCC.O.O | |
Source | PubChem | |
URL | https://pubchem.ncbi.nlm.nih.gov | |
Description | Data deposited in or computed by PubChem | |
Isomeric SMILES |
CCCCCCCCCCCCCCCCCC(=O)OC[C@H](COP(=O)([O-])OCC[N+](C)(C)C)OC(=O)CCCCCCCCCCCCCCCCC.O.O | |
Source | PubChem | |
URL | https://pubchem.ncbi.nlm.nih.gov | |
Description | Data deposited in or computed by PubChem | |
Molecular Formula |
C44H92NO10P | |
Source | PubChem | |
URL | https://pubchem.ncbi.nlm.nih.gov | |
Description | Data deposited in or computed by PubChem | |
Molecular Weight |
826.2 g/mol | |
Source | PubChem | |
URL | https://pubchem.ncbi.nlm.nih.gov | |
Description | Data deposited in or computed by PubChem | |
Haftungsausschluss und Informationen zu In-Vitro-Forschungsprodukten
Bitte beachten Sie, dass alle Artikel und Produktinformationen, die auf BenchChem präsentiert werden, ausschließlich zu Informationszwecken bestimmt sind. Die auf BenchChem zum Kauf angebotenen Produkte sind speziell für In-vitro-Studien konzipiert, die außerhalb lebender Organismen durchgeführt werden. In-vitro-Studien, abgeleitet von dem lateinischen Begriff "in Glas", beinhalten Experimente, die in kontrollierten Laborumgebungen unter Verwendung von Zellen oder Geweben durchgeführt werden. Es ist wichtig zu beachten, dass diese Produkte nicht als Arzneimittel oder Medikamente eingestuft sind und keine Zulassung der FDA für die Vorbeugung, Behandlung oder Heilung von medizinischen Zuständen, Beschwerden oder Krankheiten erhalten haben. Wir müssen betonen, dass jede Form der körperlichen Einführung dieser Produkte in Menschen oder Tiere gesetzlich strikt untersagt ist. Es ist unerlässlich, sich an diese Richtlinien zu halten, um die Einhaltung rechtlicher und ethischer Standards in Forschung und Experiment zu gewährleisten.