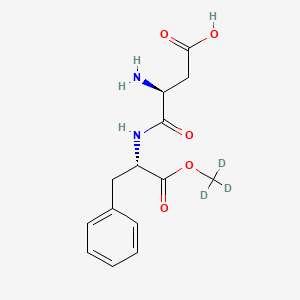
Aspartame-d3
Übersicht
Beschreibung
Aspartame is an artificial sweetener widely used in various food and beverage products since the 1980s, including diet drinks, chewing gum, gelatin, ice cream, dairy products such as yogurt, breakfast cereal, toothpaste, and medications such as cough drops and chewable vitamins .
Synthesis Analysis
Aspartame is synthesized by joining L-phenylalanine or L-phenylalanine methyl ester with L-aspartic acid . A technique designed for the simultaneous analysis of aspartame and its hydrolyzed products (amino acids such as aspartic acid and phenylalanine) has been developed .Molecular Structure Analysis
Aspartame’s structure is a combination of two amino acids, aspartic acid and phenylalanine, and can be called a dipeptide . The “natural” state of phenylalanine in aspartame has been modified by converting the acid grouping (-COOH) to an ester grouping (-COOCH3) .Chemical Reactions Analysis
The new assay for determination of aspartame was based on a set of reactions that are catalyzed by three different enzymes: α-chymotrypsin, alcohol oxidase, and horseradish peroxidase .Physical And Chemical Properties Analysis
Aspartame, as just mentioned, has ionic, polar covalent, and covalent bonding .Wissenschaftliche Forschungsanwendungen
Carcinogenic Research
Aspartame has been classified as a ‘possible carcinogen’ by the International Agency for Research on Cancer (IARC), based on limited evidence for liver cancer in studies on people and rodents . The classification is a call to the research community to better clarify and understand the carcinogenic hazard that may or may not be posed by aspartame consumption . Aspartame-d3, being a derivative of Aspartame, can be used in similar research to understand its carcinogenic properties.
Food and Beverage Industry
Aspartame is 200 times sweeter than sugar and is used in more than 6,000 products worldwide, including diet drinks, chewing gum, toothpaste, and chewable vitamins . Aspartame-d3 can be used in research to develop new low-calorie sweeteners for the food and beverage industry.
Pharmaceutical Industry
Aspartame has been linked to increased cancer risk and other health issues . Aspartame-d3 can be used in pharmaceutical research to develop drugs that can mitigate these health risks.
Biotechnological Applications
L-Aspartate dehydrogenase (L-AspDH), a member of the amino acid dehydrogenase superfamily, has potential for biotechnological applications . Aspartame-d3 can be used in research to understand the catalytic properties and physiological roles of L-AspDH.
Production of Amino Acids
L-Amino acid dehydrogenases, which include L-AspDH, are used in the production of economically important natural proteinaceous and novel non-proteinaceous amino acids for pharmaceutical industries and in medical fields . Aspartame-d3 can be used in research to improve the production of these amino acids.
Biosensors or Diagnostic Kits
L-Amino acid dehydrogenases are used in the detection and quantification of amino and keto acids, which are important tools in clinical chemistry, bioprocess controls, and nutritional studies . Aspartame-d3 can be used in research to develop new biosensors or diagnostic kits.
Wirkmechanismus
Target of Action
Aspartame-d3, like aspartame, is primarily targeted at the sweet taste receptors on the tongue . It is a low-calorie sweetener used to sweeten a wide variety of low- and reduced-calorie foods and beverages . Aspartame-d3 is composed of two amino acids, aspartic acid and phenylalanine, as the methyl ester .
Mode of Action
Aspartame-d3 interacts with its targets, the sweet taste receptors, by binding to them and triggering a response that is interpreted by the brain as a sweet taste . It is 180 to 200 times sweeter than sucrose and is metabolized as a protein, with its subsequent amino acids used up in their respective mechanisms .
Biochemical Pathways
Upon digestion, aspartame-d3 breaks down into three components: aspartic acid, phenylalanine, and methanol . These components are then absorbed into the blood and used in normal body processes . Aspartic acid and phenylalanine are also found naturally in protein-containing foods, including meats, grains, and dairy products . Methyl esters are also found naturally in many foods such as fruits and vegetables and their juices .
Pharmacokinetics
The ADME (Absorption, Distribution, Metabolism, and Excretion) properties of aspartame-d3 are similar to those of aspartame. Aspartame is hydrolyzed in the gastrointestinal tract when orally ingested . One of the major hydrolysis products is phenylalanine, which may be harmful for patients with phenylketonuria (PKU) .
Result of Action
The molecular and cellular effects of aspartame-d3’s action are similar to those of aspartame. Aspartame has the potential to impact various cancer-related proteins, potentially raising the likelihood of cellular carcinogenesis by interfering with biomolecular function . Excessive aspartame ingestion might be involved in the pathogenesis of certain mental disorders and also in compromised learning and emotional functioning .
Action Environment
Environmental factors can influence the action, efficacy, and stability of aspartame-d3. For example, the temperature and pH of the environment can affect the stability of aspartame-d3. The safety of aspartame-d3, like aspartame, is still a topic of ongoing research. The International Agency for Research on Cancer (IARC) and the World Health Organization (WHO) have released assessments of the health impacts of aspartame, citing “limited evidence” for carcinogenicity in humans .
Safety and Hazards
Assessments of the health impacts of aspartame have indicated that, while safety is not a major concern at the doses which are commonly used, potential effects have been described that need to be investigated by more and better studies . IARC classified aspartame as possibly carcinogenic to humans (Group 2B) on the basis of limited evidence for cancer in humans .
Zukünftige Richtungen
Eigenschaften
IUPAC Name |
(3S)-3-amino-4-oxo-4-[[(2S)-1-oxo-3-phenyl-1-(trideuteriomethoxy)propan-2-yl]amino]butanoic acid | |
---|---|---|
Source | PubChem | |
URL | https://pubchem.ncbi.nlm.nih.gov | |
Description | Data deposited in or computed by PubChem | |
InChI |
InChI=1S/C14H18N2O5/c1-21-14(20)11(7-9-5-3-2-4-6-9)16-13(19)10(15)8-12(17)18/h2-6,10-11H,7-8,15H2,1H3,(H,16,19)(H,17,18)/t10-,11-/m0/s1/i1D3 | |
Source | PubChem | |
URL | https://pubchem.ncbi.nlm.nih.gov | |
Description | Data deposited in or computed by PubChem | |
InChI Key |
IAOZJIPTCAWIRG-JRDZZGLNSA-N | |
Source | PubChem | |
URL | https://pubchem.ncbi.nlm.nih.gov | |
Description | Data deposited in or computed by PubChem | |
Canonical SMILES |
COC(=O)C(CC1=CC=CC=C1)NC(=O)C(CC(=O)O)N | |
Source | PubChem | |
URL | https://pubchem.ncbi.nlm.nih.gov | |
Description | Data deposited in or computed by PubChem | |
Isomeric SMILES |
[2H]C([2H])([2H])OC(=O)[C@H](CC1=CC=CC=C1)NC(=O)[C@H](CC(=O)O)N | |
Source | PubChem | |
URL | https://pubchem.ncbi.nlm.nih.gov | |
Description | Data deposited in or computed by PubChem | |
Molecular Formula |
C14H18N2O5 | |
Source | PubChem | |
URL | https://pubchem.ncbi.nlm.nih.gov | |
Description | Data deposited in or computed by PubChem | |
DSSTOX Substance ID |
DTXSID00675636 | |
Record name | (~2~H_3_)Methyl L-alpha-aspartyl-L-phenylalaninate | |
Source | EPA DSSTox | |
URL | https://comptox.epa.gov/dashboard/DTXSID00675636 | |
Description | DSSTox provides a high quality public chemistry resource for supporting improved predictive toxicology. | |
Molecular Weight |
297.32 g/mol | |
Source | PubChem | |
URL | https://pubchem.ncbi.nlm.nih.gov | |
Description | Data deposited in or computed by PubChem | |
Product Name |
Aspartame-d3 | |
CAS RN |
1356841-28-5 | |
Record name | (~2~H_3_)Methyl L-alpha-aspartyl-L-phenylalaninate | |
Source | EPA DSSTox | |
URL | https://comptox.epa.gov/dashboard/DTXSID00675636 | |
Description | DSSTox provides a high quality public chemistry resource for supporting improved predictive toxicology. | |
Haftungsausschluss und Informationen zu In-Vitro-Forschungsprodukten
Bitte beachten Sie, dass alle Artikel und Produktinformationen, die auf BenchChem präsentiert werden, ausschließlich zu Informationszwecken bestimmt sind. Die auf BenchChem zum Kauf angebotenen Produkte sind speziell für In-vitro-Studien konzipiert, die außerhalb lebender Organismen durchgeführt werden. In-vitro-Studien, abgeleitet von dem lateinischen Begriff "in Glas", beinhalten Experimente, die in kontrollierten Laborumgebungen unter Verwendung von Zellen oder Geweben durchgeführt werden. Es ist wichtig zu beachten, dass diese Produkte nicht als Arzneimittel oder Medikamente eingestuft sind und keine Zulassung der FDA für die Vorbeugung, Behandlung oder Heilung von medizinischen Zuständen, Beschwerden oder Krankheiten erhalten haben. Wir müssen betonen, dass jede Form der körperlichen Einführung dieser Produkte in Menschen oder Tiere gesetzlich strikt untersagt ist. Es ist unerlässlich, sich an diese Richtlinien zu halten, um die Einhaltung rechtlicher und ethischer Standards in Forschung und Experiment zu gewährleisten.