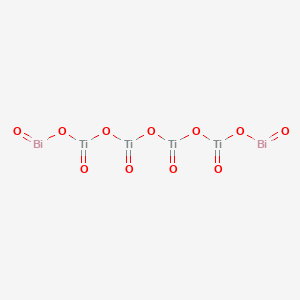
Bismuth titanate
Übersicht
Beschreibung
Bismuth titanate (Bi₄Ti₃O₁₂, BIT) is a layered Aurivillius-phase ferroelectric material composed of alternating Bi₂O₂ layers and perovskite-like (Bi₂Ti₃O₁₀)²⁻ blocks . It exhibits a high Curie temperature (650°C), making it thermally stable for high-temperature applications such as non-volatile memories, piezoelectric sensors, and microwave absorbers . BIT also demonstrates high remanent polarization (~20 μC/cm²) and low coercive fields (~50 kV/cm), which are critical for memory devices . Its orthorhombic crystal structure contributes to anisotropic optical and electrical properties, as observed in ellipsometric studies . Hydrothermal synthesis and sol-gel methods are commonly used to prepare BIT powders and thin films, ensuring high purity and controlled morphology .
Vorbereitungsmethoden
Metal-Organic Decomposition (MOD) for Thin-Film Fabrication
Synthesis Process and Precursor Layering
The alternately supplying MOD method involves sequential spin-coating of bismuth and titanium precursor layers onto silicon substrates. As demonstrated by , this approach ensures controlled stoichiometry by alternating the deposition of bismuth and titanium metal-organic precursors. The precursors are typically dissolved in solvents such as ethylene glycol or acetic acid, with spin-coating parameters (e.g., rpm, duration) tailored to achieve uniform thickness. After deposition, the films undergo a high-temperature crystallization process (600–800°C) to form the perovskite phase.
Crystallographic Orientation and Dielectric Properties
A critical finding from is the dominance of c-axis orientation in Bi₄Ti₃O₁₂ thin films when using thinner precursor layers. This orientation enhances dielectric properties, as evidenced by a dielectric constant (εᵣ) of ~220 and low leakage current in metal-insulator-semiconductor (MIS) structures. The study also notes that the order of precursor layers (Bi-first vs. Ti-first) significantly affects surface morphology, with Bi-first layers yielding smoother films.
Table 1: MOD Synthesis Parameters and Film Properties
Precursor Order | Annealing Temperature (°C) | Crystallographic Orientation | Dielectric Constant (εᵣ) |
---|---|---|---|
Bi-first | 700 | c-axis dominant | 220 ± 15 |
Ti-first | 700 | Mixed a/c-axis | 180 ± 20 |
Molten-Salt Synthesis of Platelet Morphologies
Molten-Salt Reaction Mechanism
Molten-salt synthesis, as described in and , involves reacting Bi₂O₃ and TiO₂ nanopowders in a eutectic KCl/NaCl flux at 800°C for 2 hours. The salt medium facilitates rapid diffusion and anisotropic growth, resulting in Bi₄Ti₃O₁₂ platelets with high aspect ratios (10–20 µm diameter, 200–500 nm thickness). The molar ratio of NaCl:KCl:Bi₂O₃:TiO₂ is optimized to 50:50:2:3 to ensure phase purity.
Post-Synthesis Acid Treatment and Morphological Control
Post-synthesis washing with 2 M HNO₃ removes residual salt and surface impurities, preserving platelet integrity. Transmission electron microscopy (TEM) in reveals that platelets synthesized via this method exhibit well-defined edges and minimal defects. These platelets serve as templates for heterostructures, such as SrTiO₃/Bi₄Ti₃O₁₂ nanoheterojunctions, which show enhanced photocatalytic hydrogen evolution under visible light .
Table 2: Molten-Salt Synthesis Conditions and Outcomes
Salt Composition (NaCl:KCl) | Reaction Temperature (°C) | Holding Time (h) | Platelet Dimensions | Surface Area (m²/g) |
---|---|---|---|---|
50:50 | 800 | 2 | 10–20 µm (diameter) | 8.5 ± 0.3 |
Hydrolysis Method for Nd-Doped this compound
Hydrolysis and Calcination Protocol
A patent by details the hydrolysis method for synthesizing neodymium (Nd)-doped Bi₄Ti₃O₁₂ ultrafine powders. Stoichiometric amounts of Bi(NO₃)₃·5H₂O, Nd₂O₃, and titanium butoxide (Ti(OC₄H₉)₄) are dissolved in nitric acid (pH ≤ 3), followed by hydrolysis in deionized water. Ammonia is added to precipitate the metal hydroxides, which are then calcined at 600°C for 0.5–1 hour to form Bi₄₋ₓNdₓTi₃O₁₂ (0.1 ≤ x ≤ 1.0).
Dielectric and Piezoelectric Performance
Nd doping reduces dielectric loss (tan δ < 1.0% at room temperature) and improves piezoelectric coefficients (d₃₃ ~ 25 pC/N) compared to undoped Bi₄Ti₃O₁₂. Scanning electron microscopy (SEM) in confirms that powders calcined at 750°C exhibit uniform particle sizes (100–200 nm) and high sintered density (>97%).
Table 3: Hydrolysis Method Parameters and Material Properties
Nd Doping (x) | Calcination Temperature (°C) | Particle Size (nm) | Dielectric Loss (tan δ, %) |
---|---|---|---|
0.5 | 600 | 150 ± 30 | 0.8 ± 0.1 |
0.75 | 750 | 200 ± 50 | 0.9 ± 0.2 |
Sol-Gel Synthesis for Nanopowder Production
Sol-Gel Process Optimization
Although focuses on bismuth sodium titanate (Bi₀.₅Na₀.₅TiO₃), the sol-gel methodology is adaptable to Bi₄Ti₃O₁₂. Titanium tetraisopropoxide (TTIP) and bismuth nitrate are dissolved in ethylene glycol, with nitric acid as a catalyst. Gelation occurs at 80°C, followed by calcination at 600–700°C to crystallize the perovskite phase.
Nanoparticle Morphology and Phase Purity
TEM analysis in reveals that sol-gel-derived Bi₄Ti₃O₁₂ powders calcined at 700°C for 6 hours form spherical nanoparticles (50–100 nm) with minimal agglomeration. Raman spectroscopy confirms the absence of secondary phases, such as Bi₂O₃ or TiO₂, when stoichiometric ratios are maintained.
Comparative Analysis of Synthesis Methods
Crystallinity and Morphological Trade-offs
-
MOD : Ideal for thin films but limited to small-scale substrates.
-
Molten-Salt : Produces large platelets suitable for heterostructures but requires high energy input.
-
Hydrolysis : Enables precise doping but involves complex pH control.
-
Sol-Gel : Yields nanosized powders but faces challenges in scaling up.
Dielectric and Photocatalytic Performance
Molten-sath-derived SrTiO₃/Bi₄Ti₃O₁₂ heterostructures exhibit a solar-to-hydrogen (STH) efficiency of 0.15% , while MOD-fabricated films show leakage currents below 10⁻⁷ A/cm² . Hydrolysis-synthesized Nd-doped ceramics achieve piezoelectric coefficients comparable to lead-based alternatives .
Analyse Chemischer Reaktionen
Bismuth titanate undergoes various chemical reactions, including oxidation, reduction, and substitution reactions.
Oxidation: this compound can be oxidized to form higher oxidation state compounds, such as Bi₂O₅.
Reduction: Reduction of this compound can lead to the formation of lower oxidation state compounds, such as Bi₂O₃.
Common Reagents and Conditions:
Oxidation: Oxygen or air at elevated temperatures.
Reduction: Hydrogen gas or carbon monoxide at high temperatures.
Substitution: Metal salts (e.g., lanthanum nitrate, cobalt chloride) in a suitable solvent, followed by calcination.
Major Products:
Oxidation: Bi₂O₅
Reduction: Bi₂O₃
Substitution: Doped this compound compounds with enhanced properties.
Wissenschaftliche Forschungsanwendungen
Ferroelectric and Dielectric Applications
Bismuth titanate is primarily utilized in the manufacture of capacitors and non-volatile memory devices due to its excellent dielectric properties. Its strong ferroelectric characteristics make it suitable for:
- High-Performance Capacitors : this compound capacitors exhibit high energy density and stability under varying temperatures.
- Non-Volatile Memory : The material's ability to retain polarization states enables its use in ferroelectric random access memory (FeRAM).
Case Study: Capacitor Performance
A study demonstrated that capacitors made from this compound showed a dielectric constant exceeding 1000, significantly higher than traditional materials. This property allows for miniaturization in electronic devices while maintaining performance efficiency .
Photocatalytic Applications
This compound has been modified to enhance photocatalytic activity, particularly when combined with titanium dioxide (TiO). This combination is effective in degrading organic pollutants in wastewater treatment.
- Photocatalyst Development : Research indicates that bismuth-modified TiO coatings significantly improve the degradation of various pollutants, including pesticides and pharmaceuticals. The photocatalytic activity was assessed using glass beads coated with bismuth-modified TiO, showing effective pollutant degradation with minimal loss in activity over multiple cycles .
Data Table: Pollutant Degradation Efficiency
Pollutant Type | Initial Concentration (mg/L) | Degradation Rate (%) |
---|---|---|
Herbicides | 50 | 92 |
Pesticides | 50 | 89 |
Pharmaceuticals | 50 | 85 |
Explosives | 50 | 90 |
Biomedical Applications
In the biomedical field, this compound has been explored for its potential in cancer treatment through Boron Neutron Capture Therapy (BNCT). The material's ability to absorb neutrons makes it a candidate for enhancing neutron capture efficiency.
- Neutron Capture Therapy : Studies indicate that this compound can transduce neutrons effectively, making it suitable for applications in BNCT aimed at treating superficial tumors .
Case Study: BNCT Efficacy
Research conducted at the Tehran Research Reactor demonstrated that this compound could enhance neutron flux for BNCT applications, suggesting a promising avenue for cancer treatment methodologies .
Energy Harvesting
This compound is also being investigated for energy harvesting applications due to its piezoelectric properties. It can convert mechanical energy into electrical energy, making it suitable for:
- Sensors : Used in pressure sensors and accelerometers.
- Energy Harvesting Devices : this compound-based devices can harvest energy from vibrations or mechanical stress.
Data Table: Energy Harvesting Characteristics
Property | Value |
---|---|
Piezoelectric Coefficient (d) | ~200 pC/N |
Energy Conversion Efficiency (%) | Up to 30% |
Wirkmechanismus
The mechanism of action of bismuth titanate is primarily based on its ferroelectric and photorefractive properties.
Ferroelectric Properties: this compound exhibits spontaneous polarization, which can be reversed by an external electric field.
Photorefractive Properties: this compound undergoes a reversible change in refractive index under an applied electric field or illumination.
Molecular Targets and Pathways:
Ferroelectric Pathway: Involves the alignment of electric dipoles within the crystal lattice.
Photorefractive Pathway: Involves the generation and migration of charge carriers under illumination, leading to a change in refractive index.
Vergleich Mit ähnlichen Verbindungen
Aurivillius Family Compounds
BIT belongs to the Aurivillius family, which includes:
- Strontium Bismuth Tantalate (SrBi₂Ta₂O₉, SBT) : SBT has a lower Curie temperature (~310°C) but superior fatigue resistance compared to BIT, making it suitable for FeRAM applications .
- Barium Bismuth Niobate (BaBi₂Nb₂O₉, BBN) : BBN shows higher dielectric constants (~400 at 1 kHz) than BIT (~200 at 1 kHz) but lower remanent polarization .
- Lanthanum-Doped Bismuth Titanate (Bi₃.₂₅La₀.₇₅Ti₃O₁₂, BLT) : La doping reduces leakage currents in BIT by suppressing oxygen vacancies, enhancing its suitability for thin-film capacitors .
Lead-Free Piezoelectrics
BIT is often compared to lead-free alternatives to PZT (lead zirconate titanate):
- Sodium this compound (Na₀.₅Bi₀.₅TiO₃, NBT) : NBT has a higher piezoelectric coefficient (d₃₃ ~100 pC/N) than BIT (d₃₃ ~20 pC/N) but suffers from depolarization above 200°C, limiting high-temperature use .
- Barium Titanate (BaTiO₃) : BaTiO₃ has a lower Curie temperature (~120°C) and higher d₃₃ (~190 pC/N) but is unsuitable for harsh environments .
- Potassium Sodium Niobate (K₀.₅Na₀.₅NbO₃, KNN) : KNN exhibits d₃₃ ~120 pC/N but requires complex sintering, whereas BIT’s layered structure simplifies processing .
Doped Bismuth Titanates
Doping modifies BIT’s properties for specific applications:
- Vanadium-Doped BIT (Bi₄Ti₃₋ₓVₓO₁₂) : V doping reduces electrical conductivity by 50% at x = 0.2, improving dielectric stability .
- Selenium-Substituted BIT (Bi₄Ti₂.₈Se₀.₂O₁₂) : Se substitution enhances dielectric constants (εᵣ ~220 at 1 kHz vs. ~200 for pure BIT) but lowers Curie temperatures slightly (~630°C) .
- Samarium-Doped BIT (Bi₄₋ₓSmₓTi₃O₁₂) : Sm doping increases remnant polarization to ~25 μC/cm² and reduces leakage currents in thin films .
Data Tables
Table 1: Key Properties of this compound and Similar Compounds
Table 2: Impact of Doping on this compound
Biologische Aktivität
Bismuth titanate (BiTiO, commonly referred to as BTO) is a compound that has garnered significant attention in the fields of materials science and biomedicine due to its unique properties. This article explores the biological activity of this compound, focusing on its photocatalytic capabilities, potential applications in environmental remediation, and biocompatibility.
Overview of this compound
This compound is a member of the Aurivillius family of compounds, characterized by its layered perovskite structure. It exhibits interesting electrical, optical, and photocatalytic properties, making it suitable for various applications, including photocatalysis under visible light, piezoelectric devices, and potential biomedical applications.
Photocatalytic Activity
Mechanism of Action
this compound's photocatalytic activity is primarily attributed to its narrow band gap, which allows it to absorb visible light and generate electron-hole pairs. These charge carriers can initiate redox reactions that lead to the degradation of organic pollutants.
Research Findings
- Photocatalytic Efficiency : Studies have demonstrated that this compound can effectively degrade dyes and other organic pollutants under visible light irradiation. For instance, a study reported that BiTiO exhibited photocatalytic activity with a wavelength threshold of approximately 400 nm, compared to TiO, which is only active under UV light (λ < 382 nm) .
- Comparison with Other Photocatalysts : this compound outperforms traditional TiO in terms of photocatalytic activity due to its ability to utilize a broader spectrum of sunlight . Table 1 summarizes the band gap energies and corresponding wavelengths for various this compound compositions:
Composition | Band Gap Energy (eV) | Active Wavelength (nm) |
---|---|---|
BiTiO | 3.1 | >400 |
BiTiO | 3.2 | >421 |
BiTiO | 2.5 | >496 |
Case Studies on Environmental Applications
- Dye Degradation : A study evaluated the photocatalytic degradation of methylene blue dye using synthesized this compound nanosheets. The results indicated a significant reduction in dye concentration over time when exposed to visible light, demonstrating the compound's effectiveness as a photocatalyst .
- Antimicrobial Properties : Research has shown that this compound can also eliminate microbial contaminants when utilized in photocatalytic processes. This aspect is particularly relevant for wastewater treatment applications where microbial load reduction is critical .
Biocompatibility and Biomedical Applications
This compound's biocompatibility has been investigated for potential use in biomedical applications such as drug delivery systems and tissue engineering scaffolds.
- Toxicity Studies : Preliminary studies indicate that this compound exhibits low toxicity towards human cells, making it a promising candidate for biomedical applications .
- Drug Delivery Systems : The compound's ability to facilitate controlled drug release while maintaining stability under physiological conditions has been explored, highlighting its potential in targeted therapy .
Q & A
Basic Research Questions
Q. What are the optimal hydrothermal synthesis conditions for producing phase-pure bismuth titanate (Bi₄Ti₃O₁₂)?
this compound is commonly synthesized via hydrothermal methods using Bi₂O₃ and TiO₂ precursors. Key parameters include:
- Precursor molar ratio : A 2:3 Bi:Ti ratio ensures stoichiometric Bi₄Ti₃O₁₂ formation .
- Temperature and duration : 240°C for 72 hours achieves crystallinity, with shorter durations leading to incomplete reactions .
- Alkaline medium : 3 M NaOH facilitates precursor dissolution and layered perovskite structure assembly .
XRD analysis (e.g., peaks at 2θ = 23.3°, 30.1°, 32.8°) confirms phase purity by matching ICSD standards .
Q. How does this compound’s bandgap compare to TiO₂, and what implications does this have for photocatalysis?
this compound has a narrower bandgap (2.88 eV) than TiO₂ (3.24 eV), enabling visible-light absorption. This reduces reliance on UV activation and improves photocatalytic efficiency in dye degradation (e.g., rhodamine B) . However, its layered Aurivillius structure introduces interlayer charge separation, which further mitigates electron-hole recombination .
Q. What characterization techniques are critical for validating this compound’s structural and functional properties?
- XRD : Identifies crystal phases (e.g., orthorhombic Bi₄Ti₃O₁₂ vs. cubic Bi₁₂Ti₀.₉O₁₉.₈) and lattice parameters (Table 3 in ).
- SEM/TEM : Reveals morphology and heterophase distribution .
- UV-Vis spectroscopy : Measures bandgap via Tauc plots .
- Photocatalytic assays : Quantifies degradation efficiency using Langmuir-Hinshelwood kinetics .
Advanced Research Questions
Q. How does heterophase modification (e.g., Bi₄Ti₃O₁₂/Bi₁₂Ti₀.₉O₁₉.₈) enhance photocatalytic activity in this compound?
Heterophase interfaces create electron transfer pathways between phases, reducing recombination. For example, Bi₄Ti₃O₁₂ (78.2%) and Bi₁₂Ti₀.₉O₁₉.₈ (21.8%) synergistically improve charge separation, increasing rhodamine B degradation efficiency by 120% compared to TiO₂ . Rietveld refinement of XRD data quantifies phase ratios and lattice distortions critical to this effect .
Q. What experimental strategies address contradictions in reported lattice parameters of hydrothermally synthesized this compound?
Discrepancies in lattice volume (e.g., Bi₄Ti₃O₁₂: ΔV = 0.888 ų; Bi₁₂Ti₀.₉O₁₉.₈: ΔV = 5.950 ų vs. ICSD standards) arise from synthesis conditions. To reconcile:
- Refine precursor purity : Impurities in Bi₂O₃ or TiO₂ alter crystallization .
- Adjust hydrothermal dwell time : Prolonged heating reduces lattice strain .
- Validate with multiple characterization methods : Pair XRD with Raman spectroscopy to detect subtle structural variations .
Q. Why does this compound exhibit slower initial degradation kinetics than TiO₂ despite higher overall efficiency?
While TiO₂ achieves faster initial dye adsorption due to higher surface area, this compound’s layered structure provides sustained catalytic activity via reduced recombination. For rhodamine B, TiO₂ degrades 70% in 120 minutes, but this compound achieves 98% degradation after 240 minutes with superior reusability (5% efficiency loss vs. 7% for TiO₂) . Kinetic models should account for both adsorption and charge-carrier dynamics.
Q. How can researchers optimize this compound’s synthesis for scalable photocatalytic applications?
- Scale-up hydrothermal reactors : Ensure uniform temperature/pressure to maintain phase purity .
- Dopant incorporation : Introduce transition metals (e.g., Fe³⁺) to further narrow the bandgap .
- Hybrid composites : Combine with graphene oxide to enhance electron mobility .
Q. Data Analysis and Interpretation
Q. How should researchers analyze conflicting XRD data when multiple this compound phases coexist?
- Rietveld refinement : Quantifies phase ratios and identifies secondary phases (e.g., Bi₁₂Ti₀.₉O₁₉.₈) .
- Peak deconvolution : Resolves overlapping signals (e.g., 2θ = 24.7° for cubic phase vs. 27.7° for orthorhombic) .
- Reference standards : Cross-check with ICSD entries (e.g., 98-015-9929 for Bi₄Ti₃O₁₂) .
Q. What statistical methods are appropriate for comparing photocatalytic performance across studies?
- Langmuir-Hinshelwood model : Fits first-order kinetics to degradation data .
- ANOVA : Tests significance of synthesis variables (e.g., temperature, precursor ratio) on efficiency .
- Error propagation analysis : Accounts for instrumental uncertainties in XRD and UV-Vis measurements .
Q. Ethical and Methodological Considerations
Q. How can researchers ensure reproducibility in this compound studies?
Eigenschaften
IUPAC Name |
oxo-oxobismuthanyloxy-[oxo-[oxo-(oxo(oxobismuthanyloxy)titanio)oxytitanio]oxytitanio]oxytitanium | |
---|---|---|
Source | PubChem | |
URL | https://pubchem.ncbi.nlm.nih.gov | |
Description | Data deposited in or computed by PubChem | |
InChI |
InChI=1S/2Bi.11O.4Ti | |
Source | PubChem | |
URL | https://pubchem.ncbi.nlm.nih.gov | |
Description | Data deposited in or computed by PubChem | |
InChI Key |
TZQKEPWGUIJJIY-UHFFFAOYSA-N | |
Source | PubChem | |
URL | https://pubchem.ncbi.nlm.nih.gov | |
Description | Data deposited in or computed by PubChem | |
Canonical SMILES |
O=[Ti](O[Ti](=O)O[Ti](=O)O[Bi]=O)O[Ti](=O)O[Bi]=O | |
Source | PubChem | |
URL | https://pubchem.ncbi.nlm.nih.gov | |
Description | Data deposited in or computed by PubChem | |
Molecular Formula |
Bi2O11Ti4 | |
Source | PubChem | |
URL | https://pubchem.ncbi.nlm.nih.gov | |
Description | Data deposited in or computed by PubChem | |
Molecular Weight |
785.42 g/mol | |
Source | PubChem | |
URL | https://pubchem.ncbi.nlm.nih.gov | |
Description | Data deposited in or computed by PubChem | |
Haftungsausschluss und Informationen zu In-Vitro-Forschungsprodukten
Bitte beachten Sie, dass alle Artikel und Produktinformationen, die auf BenchChem präsentiert werden, ausschließlich zu Informationszwecken bestimmt sind. Die auf BenchChem zum Kauf angebotenen Produkte sind speziell für In-vitro-Studien konzipiert, die außerhalb lebender Organismen durchgeführt werden. In-vitro-Studien, abgeleitet von dem lateinischen Begriff "in Glas", beinhalten Experimente, die in kontrollierten Laborumgebungen unter Verwendung von Zellen oder Geweben durchgeführt werden. Es ist wichtig zu beachten, dass diese Produkte nicht als Arzneimittel oder Medikamente eingestuft sind und keine Zulassung der FDA für die Vorbeugung, Behandlung oder Heilung von medizinischen Zuständen, Beschwerden oder Krankheiten erhalten haben. Wir müssen betonen, dass jede Form der körperlichen Einführung dieser Produkte in Menschen oder Tiere gesetzlich strikt untersagt ist. Es ist unerlässlich, sich an diese Richtlinien zu halten, um die Einhaltung rechtlicher und ethischer Standards in Forschung und Experiment zu gewährleisten.