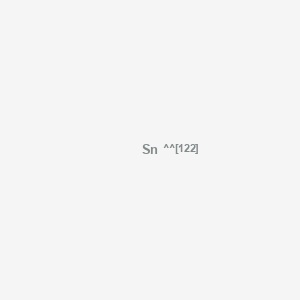
tin-122
- Klicken Sie auf QUICK INQUIRY, um ein Angebot von unserem Expertenteam zu erhalten.
- Mit qualitativ hochwertigen Produkten zu einem WETTBEWERBSFÄHIGEN Preis können Sie sich mehr auf Ihre Forschung konzentrieren.
Übersicht
Beschreibung
Tin-122, also known as 122-Sn, is an isotope of tin with an atomic number of 50 and a mass number of 122. It is a stable isotope, meaning it does not undergo radioactive decay. This compound is one of the naturally occurring isotopes of tin, which is a soft, malleable metal known for its resistance to corrosion .
Vorbereitungsmethoden
The preparation of tin-122 typically involves the separation of tin isotopes from naturally occurring tin. This can be achieved through various methods such as gas centrifugation or electromagnetic separation. Industrial production of this compound may involve the use of advanced techniques like laser isotope separation, which allows for the precise extraction of specific isotopes from a mixture .
Analyse Chemischer Reaktionen
Tin-122, like other tin isotopes, can undergo various chemical reactions. Some of the common types of reactions include:
Oxidation: Tin can react with oxygen to form tin oxides. For example, when heated in the presence of oxygen, tin can form tin(IV) oxide (SnO₂).
Reduction: Tin can be reduced from its oxides using reducing agents like hydrogen or carbon.
Substitution: Tin can participate in substitution reactions, where it replaces another element in a compound. .
Wissenschaftliche Forschungsanwendungen
Tin-122 has several applications in scientific research, including:
Chemistry: this compound is used in the study of tin compounds and their reactions. It is also used in the synthesis of various tin-based materials.
Biology: Tin compounds, including those containing this compound, are studied for their potential biological activities, such as antimicrobial properties.
Medicine: this compound is used in the development of radiopharmaceuticals for medical imaging and diagnostic purposes.
Industry: This compound is used in the production of tin-based alloys and coatings, which are valued for their corrosion resistance and other desirable properties .
Wirkmechanismus
The mechanism of action of tin-122 depends on the specific compound it is part of. For example, in radiopharmaceuticals, this compound can act as a reducing agent, facilitating the formation of complexes with other elements. These complexes can then be used for imaging or therapeutic purposes. The molecular targets and pathways involved vary depending on the specific application and compound .
Vergleich Mit ähnlichen Verbindungen
Tin-122 can be compared with other isotopes of tin, such as tin-118, tin-119, and tin-120. Each isotope has unique properties and applications:
Tin-118: Used in the study of nuclear reactions and as a tracer in various scientific experiments.
Tin-119: Known for its use in Mössbauer spectroscopy, a technique used to study the properties of materials.
Tin-120: Used in the production of tin-based alloys and in the study of nuclear physics.
This compound is unique in its stability and its specific applications in scientific research and industry. Its ability to form stable compounds and participate in various chemical reactions makes it a valuable isotope for a wide range of applications.
Eigenschaften
CAS-Nummer |
14119-18-7 |
---|---|
Molekularformel |
Sn |
Molekulargewicht |
121.903 |
IUPAC-Name |
tin-122 |
InChI |
InChI=1S/Sn/i1+3 |
InChI-Schlüssel |
ATJFFYVFTNAWJD-AKLPVKDBSA-N |
SMILES |
[Sn] |
Herkunft des Produkts |
United States |
Haftungsausschluss und Informationen zu In-Vitro-Forschungsprodukten
Bitte beachten Sie, dass alle Artikel und Produktinformationen, die auf BenchChem präsentiert werden, ausschließlich zu Informationszwecken bestimmt sind. Die auf BenchChem zum Kauf angebotenen Produkte sind speziell für In-vitro-Studien konzipiert, die außerhalb lebender Organismen durchgeführt werden. In-vitro-Studien, abgeleitet von dem lateinischen Begriff "in Glas", beinhalten Experimente, die in kontrollierten Laborumgebungen unter Verwendung von Zellen oder Geweben durchgeführt werden. Es ist wichtig zu beachten, dass diese Produkte nicht als Arzneimittel oder Medikamente eingestuft sind und keine Zulassung der FDA für die Vorbeugung, Behandlung oder Heilung von medizinischen Zuständen, Beschwerden oder Krankheiten erhalten haben. Wir müssen betonen, dass jede Form der körperlichen Einführung dieser Produkte in Menschen oder Tiere gesetzlich strikt untersagt ist. Es ist unerlässlich, sich an diese Richtlinien zu halten, um die Einhaltung rechtlicher und ethischer Standards in Forschung und Experiment zu gewährleisten.