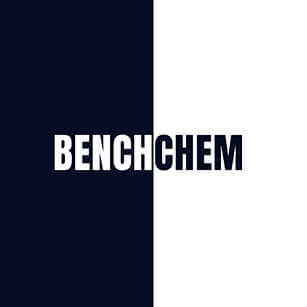
(3R,4S)-4-phenyloxolane-3-carboxylic acid
- Klicken Sie auf QUICK INQUIRY, um ein Angebot von unserem Expertenteam zu erhalten.
- Mit qualitativ hochwertigen Produkten zu einem WETTBEWERBSFÄHIGEN Preis können Sie sich mehr auf Ihre Forschung konzentrieren.
Übersicht
Beschreibung
(3R,4S)-4-Phenyloxolane-3-carboxylic acid is a chiral compound with significant importance in organic chemistry. It features a five-membered oxolane ring with a phenyl group attached to the fourth carbon and a carboxylic acid group at the third carbon. The stereochemistry of this compound is defined by the (3R,4S) configuration, which influences its reactivity and interaction with other molecules.
Vorbereitungsmethoden
Synthetic Routes and Reaction Conditions: The synthesis of (3R,4S)-4-phenyloxolane-3-carboxylic acid typically involves the following steps:
Epoxidation of Alkenes: The initial step often involves the epoxidation of an alkene precursor using peroxy acids such as meta-chloroperoxybenzoic acid (mCPBA) or peroxyacetic acid.
Ring-Opening Reactions: The resulting epoxide can undergo ring-opening reactions under acidic or basic conditions to form the oxolane ring.
Stereoselective Reactions: The stereochemistry is controlled through the use of chiral catalysts or reagents to ensure the (3R,4S) configuration.
Industrial Production Methods: Industrial production of this compound may involve large-scale epoxidation and ring-opening processes, utilizing continuous flow reactors to maintain consistent reaction conditions and high yields .
Types of Reactions:
Reduction: Reduction reactions can target the carboxylic acid group, converting it to an alcohol or aldehyde.
Substitution: Nucleophilic substitution reactions can occur at the oxolane ring, especially under acidic or basic conditions.
Common Reagents and Conditions:
Oxidation: Common oxidizing agents include potassium permanganate (KMnO₄) and chromium trioxide (CrO₃).
Reduction: Reducing agents such as lithium aluminum hydride (LiAlH₄) and sodium borohydride (NaBH₄) are frequently used.
Substitution: Acidic conditions (e.g., HCl) or basic conditions (e.g., NaOH) facilitate substitution reactions.
Major Products:
Oxidation: Phenolic derivatives.
Reduction: Alcohols or aldehydes.
Substitution: Various substituted oxolane derivatives.
Wissenschaftliche Forschungsanwendungen
(3R,4S)-4-Phenyloxolane-3-carboxylic acid has diverse applications in scientific research:
Wirkmechanismus
The mechanism of action of (3R,4S)-4-phenyloxolane-3-carboxylic acid involves its interaction with specific molecular targets, such as enzymes or receptors. The (3R,4S) configuration plays a crucial role in determining the binding affinity and specificity of the compound . The oxolane ring and phenyl group contribute to the overall molecular recognition and interaction with biological targets .
Vergleich Mit ähnlichen Verbindungen
Pyrrolidine Derivatives: Compounds like pyrrolidine-2,5-diones and prolinol share structural similarities with (3R,4S)-4-phenyloxolane-3-carboxylic acid.
Homoisoflavonoids: These compounds, such as sappanol and episappanol, also feature a five-membered ring structure with phenyl substitutions.
Uniqueness: this compound is unique due to its specific stereochemistry and the presence of both an oxolane ring and a carboxylic acid group. This combination imparts distinct reactivity and interaction profiles compared to other similar compounds .
Biologische Aktivität
(3R,4S)-4-phenyloxolane-3-carboxylic acid is a compound of interest due to its potential biological activities. This article reviews the current understanding of its biological properties, including antimicrobial, anti-inflammatory, and cytotoxic effects. The findings are supported by data tables and case studies from various research sources.
Chemical Structure
The molecular structure of this compound can be represented as follows:
- Molecular Formula : C₁₁H₁₂O₃
- Molecular Weight : 192.21 g/mol
Antimicrobial Activity
Several studies have investigated the antimicrobial properties of this compound. Notably, it has shown effectiveness against various bacterial strains.
Microorganism | Minimum Inhibitory Concentration (MIC) |
---|---|
Staphylococcus aureus | 12.5 μg/mL |
Methicillin-resistant Staphylococcus aureus (MRSA) | 10.0 μg/mL |
Escherichia coli | 31.25 μg/mL |
These results indicate that the compound exhibits significant antimicrobial activity, particularly against Gram-positive bacteria, which are often resistant to conventional antibiotics .
Anti-inflammatory Effects
Research has highlighted the potential anti-inflammatory effects of this compound. It was observed to inhibit the expression of pro-inflammatory cytokines in cell cultures.
- Key Findings :
- Inhibition of nitric oxide production in murine microglial cells.
- Reduction in cyclooxygenase-2 (COX-2) expression with an IC50 value of 16.6 μM.
These findings suggest that the compound may have therapeutic potential in treating inflammatory diseases .
Cytotoxicity
Cytotoxicity assays have revealed that this compound has selective cytotoxic effects on cancer cell lines.
Cell Line | IC50 Value |
---|---|
Human breast cancer MCF-7 | 15 μM |
Human lung cancer NCI-H187 | 6.7 μg/mL |
This data indicates that the compound could be a candidate for further development as an anticancer agent .
Case Studies
-
Study on Antimicrobial Activity :
A recent study isolated this compound from Xylaria sp. and tested its efficacy against various pathogens. The results showed promising antimicrobial properties with low MIC values against key bacterial strains. -
Study on Anti-inflammatory Properties :
In vitro experiments demonstrated that the compound significantly reduced the production of inflammatory markers in stimulated macrophages, suggesting its potential use in managing chronic inflammatory conditions.
Eigenschaften
CAS-Nummer |
1268521-40-9 |
---|---|
Molekularformel |
C11H12O3 |
Molekulargewicht |
192.21 g/mol |
IUPAC-Name |
(3R,4S)-4-phenyloxolane-3-carboxylic acid |
InChI |
InChI=1S/C11H12O3/c12-11(13)10-7-14-6-9(10)8-4-2-1-3-5-8/h1-5,9-10H,6-7H2,(H,12,13)/t9-,10+/m1/s1 |
InChI-Schlüssel |
RUOOGZAGKZOJQK-ZJUUUORDSA-N |
SMILES |
C1C(C(CO1)C(=O)O)C2=CC=CC=C2 |
Isomerische SMILES |
C1[C@@H]([C@H](CO1)C(=O)O)C2=CC=CC=C2 |
Kanonische SMILES |
C1C(C(CO1)C(=O)O)C2=CC=CC=C2 |
Synonyme |
(3R,4S)-4-phenyloxolane-3-carboxylic acid |
Herkunft des Produkts |
United States |
Haftungsausschluss und Informationen zu In-Vitro-Forschungsprodukten
Bitte beachten Sie, dass alle Artikel und Produktinformationen, die auf BenchChem präsentiert werden, ausschließlich zu Informationszwecken bestimmt sind. Die auf BenchChem zum Kauf angebotenen Produkte sind speziell für In-vitro-Studien konzipiert, die außerhalb lebender Organismen durchgeführt werden. In-vitro-Studien, abgeleitet von dem lateinischen Begriff "in Glas", beinhalten Experimente, die in kontrollierten Laborumgebungen unter Verwendung von Zellen oder Geweben durchgeführt werden. Es ist wichtig zu beachten, dass diese Produkte nicht als Arzneimittel oder Medikamente eingestuft sind und keine Zulassung der FDA für die Vorbeugung, Behandlung oder Heilung von medizinischen Zuständen, Beschwerden oder Krankheiten erhalten haben. Wir müssen betonen, dass jede Form der körperlichen Einführung dieser Produkte in Menschen oder Tiere gesetzlich strikt untersagt ist. Es ist unerlässlich, sich an diese Richtlinien zu halten, um die Einhaltung rechtlicher und ethischer Standards in Forschung und Experiment zu gewährleisten.