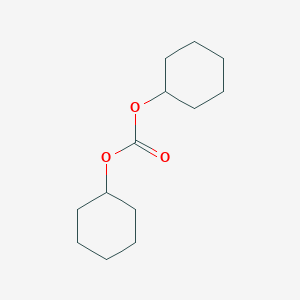
Dicyclohexyl carbonate
Übersicht
Beschreibung
Dicyclohexyl carbonate is an organic compound with the chemical formula C₁₄H₂₂O₃. It is a white crystalline solid that is used in various chemical reactions and industrial applications. This compound is known for its role as a reagent in organic synthesis, particularly in the formation of esters and carbonates.
Wissenschaftliche Forschungsanwendungen
Dicyclohexyl carbonate is used in various scientific research applications, including:
Organic Synthesis: Used as a reagent in the synthesis of esters and carbonates.
Polymer Chemistry: Employed in the production of polycarbonates and polyesters.
Pharmaceuticals: Utilized in the synthesis of active pharmaceutical ingredients.
Material Science: Applied in the development of advanced materials with specific properties.
Safety and Hazards
Dicyclohexyl carbonate should be handled with care. Avoid dust formation, breathing mist, gas or vapours, and contact with skin and eye. Use personal protective equipment, wear chemical impermeable gloves, ensure adequate ventilation, remove all sources of ignition, evacuate personnel to safe areas, and keep people away from and upwind of spill/leak .
Wirkmechanismus
Target of Action
Dicyclohexyl carbonate, also known as N,N’-Dicyclohexylcarbodiimide (DCC), is primarily used as a coupling agent in the synthesis of peptides . Its primary targets are amino acids, specifically their carboxyl and amino groups .
Mode of Action
DCC acts as a dehydrating agent, facilitating the formation of amide bonds between amino acids during peptide synthesis . It does this by reacting with the carboxyl group of one amino acid and the amino group of another, leading to the formation of a peptide bond and the release of dicyclohexylurea (DCU) as a byproduct .
Biochemical Pathways
The primary biochemical pathway affected by DCC is peptide synthesis. In this process, DCC facilitates the formation of peptide bonds, which are the links between individual amino acids in a peptide or protein . The byproduct of this reaction, DCU, is insoluble in most organic solvents, making it easy to remove from the reaction mixture .
Pharmacokinetics
Information on the ADME (Absorption, Distribution, Metabolism, and Excretion) properties of DCC is limited. It is known that dcc is highly soluble in organic solvents such as dichloromethane, tetrahydrofuran, acetonitrile, and dimethylformamide, but insoluble in water . This suggests that its bioavailability may be influenced by these properties.
Result of Action
The primary result of DCC’s action is the formation of peptide bonds, leading to the synthesis of peptides or proteins . This is crucial in biological systems, as proteins perform a vast array of functions, including catalyzing metabolic reactions, DNA replication, responding to stimuli, and transporting molecules from one location to another.
Action Environment
DCC is sensitive to moisture and needs to be handled under anhydrous conditions . It is typically used in dry or anhydrous environments due to its sensitivity to moisture . Environmental factors such as temperature and pH could potentially influence the efficacy and stability of DCC, but specific details would depend on the exact conditions of the reaction it’s being used in.
Vorbereitungsmethoden
Synthetic Routes and Reaction Conditions
Dicyclohexyl carbonate can be synthesized through several methods. One common method involves the reaction of cyclohexanol with phosgene in the presence of a base. The reaction proceeds as follows:
2C6H11OH+COCl2→(C6H11O)2CO+2HCl
This reaction requires careful handling of phosgene, a toxic and hazardous reagent.
Another method involves the transesterification of dimethyl carbonate with cyclohexanol. This reaction is catalyzed by a base such as sodium methoxide:
CH3OCOOCH3+2C6H11OH→(C6H11O)2CO+2CH3OH
Industrial Production Methods
In industrial settings, this compound is often produced using the transesterification method due to its relatively safer and more environmentally friendly process compared to the use of phosgene. The reaction is typically carried out in large reactors with continuous removal of methanol to drive the reaction to completion.
Analyse Chemischer Reaktionen
Types of Reactions
Dicyclohexyl carbonate undergoes various chemical reactions, including:
Esterification: Reacts with alcohols to form esters.
Transesterification: Exchanges the alkoxy group with another alcohol.
Hydrolysis: Reacts with water to form cyclohexanol and carbon dioxide.
Common Reagents and Conditions
Bases: Sodium methoxide, potassium carbonate.
Acids: Sulfuric acid, hydrochloric acid.
Solvents: Toluene, dichloromethane, acetonitrile.
Major Products
Esters: Formed through esterification reactions.
Alcohols: Produced during hydrolysis and transesterification reactions.
Vergleich Mit ähnlichen Verbindungen
Similar Compounds
Dimethyl Carbonate: A similar carbonate compound used in transesterification reactions.
Diethyl Carbonate: Another carbonate used in organic synthesis.
Diphenyl Carbonate: Used in the production of polycarbonates.
Uniqueness
Dicyclohexyl carbonate is unique due to its higher molecular weight and bulkier structure compared to dimethyl carbonate and diethyl carbonate. This makes it more suitable for specific applications where steric hindrance is beneficial, such as in the synthesis of certain polymers and advanced materials.
Eigenschaften
IUPAC Name |
dicyclohexyl carbonate | |
---|---|---|
Source | PubChem | |
URL | https://pubchem.ncbi.nlm.nih.gov | |
Description | Data deposited in or computed by PubChem | |
InChI |
InChI=1S/C13H22O3/c14-13(15-11-7-3-1-4-8-11)16-12-9-5-2-6-10-12/h11-12H,1-10H2 | |
Source | PubChem | |
URL | https://pubchem.ncbi.nlm.nih.gov | |
Description | Data deposited in or computed by PubChem | |
InChI Key |
FYIBPWZEZWVDQB-UHFFFAOYSA-N | |
Source | PubChem | |
URL | https://pubchem.ncbi.nlm.nih.gov | |
Description | Data deposited in or computed by PubChem | |
Canonical SMILES |
C1CCC(CC1)OC(=O)OC2CCCCC2 | |
Source | PubChem | |
URL | https://pubchem.ncbi.nlm.nih.gov | |
Description | Data deposited in or computed by PubChem | |
Molecular Formula |
C13H22O3 | |
Source | PubChem | |
URL | https://pubchem.ncbi.nlm.nih.gov | |
Description | Data deposited in or computed by PubChem | |
DSSTOX Substance ID |
DTXSID60196099 | |
Record name | Carbonic acid, dicyclohexyl ester | |
Source | EPA DSSTox | |
URL | https://comptox.epa.gov/dashboard/DTXSID60196099 | |
Description | DSSTox provides a high quality public chemistry resource for supporting improved predictive toxicology. | |
Molecular Weight |
226.31 g/mol | |
Source | PubChem | |
URL | https://pubchem.ncbi.nlm.nih.gov | |
Description | Data deposited in or computed by PubChem | |
CAS No. |
4427-97-8 | |
Record name | Dicyclohexyl carbonate | |
Source | CAS Common Chemistry | |
URL | https://commonchemistry.cas.org/detail?cas_rn=4427-97-8 | |
Description | CAS Common Chemistry is an open community resource for accessing chemical information. Nearly 500,000 chemical substances from CAS REGISTRY cover areas of community interest, including common and frequently regulated chemicals, and those relevant to high school and undergraduate chemistry classes. This chemical information, curated by our expert scientists, is provided in alignment with our mission as a division of the American Chemical Society. | |
Explanation | The data from CAS Common Chemistry is provided under a CC-BY-NC 4.0 license, unless otherwise stated. | |
Record name | Carbonic acid, dicyclohexyl ester | |
Source | ChemIDplus | |
URL | https://pubchem.ncbi.nlm.nih.gov/substance/?source=chemidplus&sourceid=0004427978 | |
Description | ChemIDplus is a free, web search system that provides access to the structure and nomenclature authority files used for the identification of chemical substances cited in National Library of Medicine (NLM) databases, including the TOXNET system. | |
Record name | Carbonic acid, dicyclohexyl ester | |
Source | EPA DSSTox | |
URL | https://comptox.epa.gov/dashboard/DTXSID60196099 | |
Description | DSSTox provides a high quality public chemistry resource for supporting improved predictive toxicology. | |
Retrosynthesis Analysis
AI-Powered Synthesis Planning: Our tool employs the Template_relevance Pistachio, Template_relevance Bkms_metabolic, Template_relevance Pistachio_ringbreaker, Template_relevance Reaxys, Template_relevance Reaxys_biocatalysis model, leveraging a vast database of chemical reactions to predict feasible synthetic routes.
One-Step Synthesis Focus: Specifically designed for one-step synthesis, it provides concise and direct routes for your target compounds, streamlining the synthesis process.
Accurate Predictions: Utilizing the extensive PISTACHIO, BKMS_METABOLIC, PISTACHIO_RINGBREAKER, REAXYS, REAXYS_BIOCATALYSIS database, our tool offers high-accuracy predictions, reflecting the latest in chemical research and data.
Strategy Settings
Precursor scoring | Relevance Heuristic |
---|---|
Min. plausibility | 0.01 |
Model | Template_relevance |
Template Set | Pistachio/Bkms_metabolic/Pistachio_ringbreaker/Reaxys/Reaxys_biocatalysis |
Top-N result to add to graph | 6 |
Feasible Synthetic Routes
Haftungsausschluss und Informationen zu In-Vitro-Forschungsprodukten
Bitte beachten Sie, dass alle Artikel und Produktinformationen, die auf BenchChem präsentiert werden, ausschließlich zu Informationszwecken bestimmt sind. Die auf BenchChem zum Kauf angebotenen Produkte sind speziell für In-vitro-Studien konzipiert, die außerhalb lebender Organismen durchgeführt werden. In-vitro-Studien, abgeleitet von dem lateinischen Begriff "in Glas", beinhalten Experimente, die in kontrollierten Laborumgebungen unter Verwendung von Zellen oder Geweben durchgeführt werden. Es ist wichtig zu beachten, dass diese Produkte nicht als Arzneimittel oder Medikamente eingestuft sind und keine Zulassung der FDA für die Vorbeugung, Behandlung oder Heilung von medizinischen Zuständen, Beschwerden oder Krankheiten erhalten haben. Wir müssen betonen, dass jede Form der körperlichen Einführung dieser Produkte in Menschen oder Tiere gesetzlich strikt untersagt ist. Es ist unerlässlich, sich an diese Richtlinien zu halten, um die Einhaltung rechtlicher und ethischer Standards in Forschung und Experiment zu gewährleisten.