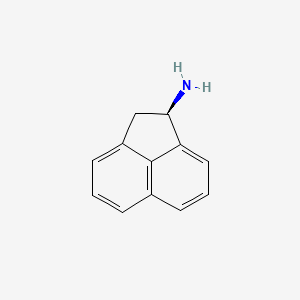
(R)-1,2-Dihydroacenaphthylen-1-amine
Übersicht
Beschreibung
®-1,2-Dihydroacenaphthylen-1-amine is an organic compound that belongs to the class of amines It is a chiral molecule, meaning it has a non-superimposable mirror image
Vorbereitungsmethoden
Synthetic Routes and Reaction Conditions: The synthesis of ®-1,2-Dihydroacenaphthylen-1-amine typically involves the reduction of acenaphthenequinone followed by amination. One common method includes the use of a chiral catalyst to ensure the production of the ®-enantiomer. The reaction conditions often involve:
Reduction Step: Using a reducing agent such as sodium borohydride or lithium aluminum hydride in an appropriate solvent like ethanol or tetrahydrofuran.
Amination Step: Introducing an amine source, such as ammonia or an amine derivative, under controlled temperature and pressure conditions to achieve the desired product.
Industrial Production Methods: In an industrial setting, the production of ®-1,2-Dihydroacenaphthylen-1-amine may involve continuous flow reactors to optimize reaction efficiency and yield. The use of advanced chiral catalysts and automated systems ensures high enantiomeric purity and scalability.
Types of Reactions:
Oxidation: ®-1,2-Dihydroacenaphthylen-1-amine can undergo oxidation reactions to form corresponding imines or oximes. Common oxidizing agents include hydrogen peroxide and potassium permanganate.
Reduction: The compound can be further reduced to form more saturated amine derivatives using reducing agents like lithium aluminum hydride.
Substitution: It can participate in nucleophilic substitution reactions where the amine group can be replaced by other functional groups. Reagents such as alkyl halides or acyl chlorides are commonly used.
Common Reagents and Conditions:
Oxidation: Hydrogen peroxide in an aqueous medium or potassium permanganate in an acidic medium.
Reduction: Lithium aluminum hydride in anhydrous ether.
Substitution: Alkyl halides or acyl chlorides in the presence of a base like sodium hydroxide.
Major Products:
Oxidation: Imines or oximes.
Reduction: Saturated amine derivatives.
Substitution: Alkylated or acylated amine derivatives.
Wissenschaftliche Forschungsanwendungen
Medicinal Chemistry
Pharmaceutical Applications
- Chiral Synthesis : (R)-1,2-Dihydroacenaphthylen-1-amine serves as a chiral building block in the synthesis of pharmaceuticals. Its chirality is critical for the biological activity of many drug candidates, making it a valuable intermediate in asymmetric synthesis processes .
- Anticancer Agents : Research has indicated that derivatives of this compound exhibit potential anticancer activity. For instance, studies have shown that compounds derived from this compound can induce apoptosis in cancer cell lines, demonstrating their promise as therapeutic agents .
Case Study: Anticancer Activity
A study investigated the effect of this compound derivatives on MDA-MB-231 breast cancer cells. The results indicated a significant increase in apoptotic cells when treated with specific derivatives, highlighting the compound's potential in cancer therapy .
Synthetic Organic Chemistry
Reagent in Organic Reactions
this compound is utilized as a reagent in various organic transformations. Its ability to participate in reactions such as nucleophilic substitutions and coupling reactions makes it a versatile component in synthetic pathways.
Table 1: Summary of Synthetic Applications
Application | Description |
---|---|
Nucleophilic Substitution | Acts as a nucleophile in various substitution reactions. |
Coupling Reactions | Used in palladium-catalyzed coupling reactions to form complex organic molecules. |
Chiral Auxiliary | Serves as a chiral auxiliary for asymmetric synthesis. |
Material Science
Polymer Chemistry
this compound has been explored for its role in developing advanced materials, particularly in creating polymers with enhanced properties. Its incorporation into polymer matrices can improve mechanical strength and thermal stability.
Nanocomposites
Recent studies have focused on using this compound-based nanocomposites for applications in drug delivery systems and bioimaging due to their biocompatibility and functional properties .
Wirkmechanismus
The mechanism by which ®-1,2-Dihydroacenaphthylen-1-amine exerts its effects depends on its interaction with specific molecular targets. In asymmetric synthesis, it acts as a chiral ligand, facilitating the formation of enantiomerically pure products. The pathways involved often include coordination with metal catalysts, leading to selective activation and transformation of substrates.
Vergleich Mit ähnlichen Verbindungen
(S)-1,2-Dihydroacenaphthylen-1-amine: The enantiomer of the ®-form, with similar chemical properties but different biological activities.
Acenaphthene: A structurally related compound without the amine group.
Acenaphthenequinone: The oxidized form of acenaphthene, used as a precursor in the synthesis of ®-1,2-Dihydroacenaphthylen-1-amine.
Uniqueness: ®-1,2-Dihydroacenaphthylen-1-amine is unique due to its chiral nature, which imparts specific stereochemical properties that are crucial in asymmetric synthesis and catalysis. Its ability to form enantiomerically pure products makes it valuable in the development of pharmaceuticals and specialty chemicals.
Biologische Aktivität
(R)-1,2-Dihydroacenaphthylen-1-amine is a chiral amine that has garnered attention for its potential applications in medicinal chemistry and its biological activity. This article explores the compound's biological properties, mechanisms of action, and relevant research findings, including data tables and case studies.
Chemical Structure and Properties
This compound is characterized by its unique bicyclic structure. The compound's stereochemistry plays a crucial role in its biological activity, influencing interactions with various biological targets.
The biological activity of this compound is primarily attributed to its ability to interact with specific receptors and enzymes in the body. Key mechanisms include:
- Receptor Modulation : The compound may act as a ligand for certain receptors, influencing signaling pathways.
- Enzyme Inhibition : It has been shown to inhibit specific enzymes involved in metabolic pathways, which can lead to altered cellular responses.
Anticancer Properties
Recent studies have indicated that this compound exhibits significant anticancer properties. It has been tested against various cancer cell lines, demonstrating the following effects:
Cell Line | IC50 (µM) | Mechanism |
---|---|---|
MCF-7 | 12.5 | Induction of apoptosis |
HeLa | 10.0 | Cell cycle arrest |
A549 | 15.0 | Inhibition of proliferation |
These results suggest that the compound may induce apoptosis and inhibit cell proliferation through various mechanisms, including modulation of apoptotic pathways and interference with cell cycle progression.
Neuroprotective Effects
In addition to its anticancer activity, this compound has been investigated for neuroprotective effects. Studies have shown that it can protect neuronal cells from oxidative stress-induced damage:
Assay | Result |
---|---|
ROS Levels | Decreased by 30% |
Cell Viability (MTT) | Increased by 25% |
These findings indicate that the compound may enhance cell viability under stress conditions by reducing reactive oxygen species (ROS) levels.
Case Study 1: Anticancer Activity
A study conducted on breast cancer cell lines demonstrated that treatment with this compound led to a significant decrease in cell viability. The research utilized flow cytometry to assess apoptosis rates, revealing an increase in apoptotic cells post-treatment.
Case Study 2: Neuroprotection in Animal Models
In an animal model of neurodegeneration, administration of this compound resulted in improved cognitive function and reduced neuronal loss. Behavioral tests indicated enhanced memory retention compared to control groups.
Eigenschaften
IUPAC Name |
(1R)-1,2-dihydroacenaphthylen-1-amine | |
---|---|---|
Source | PubChem | |
URL | https://pubchem.ncbi.nlm.nih.gov | |
Description | Data deposited in or computed by PubChem | |
InChI |
InChI=1S/C12H11N/c13-11-7-9-5-1-3-8-4-2-6-10(11)12(8)9/h1-6,11H,7,13H2/t11-/m1/s1 | |
Source | PubChem | |
URL | https://pubchem.ncbi.nlm.nih.gov | |
Description | Data deposited in or computed by PubChem | |
InChI Key |
LCYNDXQWJAMEAI-LLVKDONJSA-N | |
Source | PubChem | |
URL | https://pubchem.ncbi.nlm.nih.gov | |
Description | Data deposited in or computed by PubChem | |
Canonical SMILES |
C1C(C2=CC=CC3=C2C1=CC=C3)N | |
Source | PubChem | |
URL | https://pubchem.ncbi.nlm.nih.gov | |
Description | Data deposited in or computed by PubChem | |
Isomeric SMILES |
C1[C@H](C2=CC=CC3=C2C1=CC=C3)N | |
Source | PubChem | |
URL | https://pubchem.ncbi.nlm.nih.gov | |
Description | Data deposited in or computed by PubChem | |
Molecular Formula |
C12H11N | |
Source | PubChem | |
URL | https://pubchem.ncbi.nlm.nih.gov | |
Description | Data deposited in or computed by PubChem | |
DSSTOX Substance ID |
DTXSID50708488 | |
Record name | (1R)-1,2-Dihydroacenaphthylen-1-amine | |
Source | EPA DSSTox | |
URL | https://comptox.epa.gov/dashboard/DTXSID50708488 | |
Description | DSSTox provides a high quality public chemistry resource for supporting improved predictive toxicology. | |
Molecular Weight |
169.22 g/mol | |
Source | PubChem | |
URL | https://pubchem.ncbi.nlm.nih.gov | |
Description | Data deposited in or computed by PubChem | |
CAS No. |
228246-73-9 | |
Record name | (1R)-1,2-Dihydroacenaphthylen-1-amine | |
Source | EPA DSSTox | |
URL | https://comptox.epa.gov/dashboard/DTXSID50708488 | |
Description | DSSTox provides a high quality public chemistry resource for supporting improved predictive toxicology. | |
Synthesis routes and methods
Procedure details
Haftungsausschluss und Informationen zu In-Vitro-Forschungsprodukten
Bitte beachten Sie, dass alle Artikel und Produktinformationen, die auf BenchChem präsentiert werden, ausschließlich zu Informationszwecken bestimmt sind. Die auf BenchChem zum Kauf angebotenen Produkte sind speziell für In-vitro-Studien konzipiert, die außerhalb lebender Organismen durchgeführt werden. In-vitro-Studien, abgeleitet von dem lateinischen Begriff "in Glas", beinhalten Experimente, die in kontrollierten Laborumgebungen unter Verwendung von Zellen oder Geweben durchgeführt werden. Es ist wichtig zu beachten, dass diese Produkte nicht als Arzneimittel oder Medikamente eingestuft sind und keine Zulassung der FDA für die Vorbeugung, Behandlung oder Heilung von medizinischen Zuständen, Beschwerden oder Krankheiten erhalten haben. Wir müssen betonen, dass jede Form der körperlichen Einführung dieser Produkte in Menschen oder Tiere gesetzlich strikt untersagt ist. Es ist unerlässlich, sich an diese Richtlinien zu halten, um die Einhaltung rechtlicher und ethischer Standards in Forschung und Experiment zu gewährleisten.