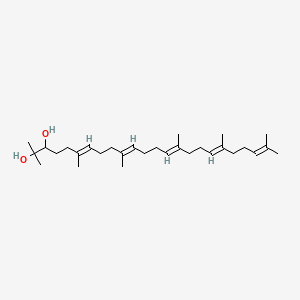
Squalene-2,3-diol
Übersicht
Beschreibung
Squalene-2,3-diol (CAS: 14031-37-9) is a triterpenoid derivative with the molecular formula C₃₀H₅₂O₂ and a molecular weight of 444.733 g/mol . It is a dihydroxylated analog of squalene, a key intermediate in the biosynthesis of sterols and triterpenoids. Structurally, this compound features two hydroxyl groups at positions 2 and 3 of the squalene backbone, distinguishing it from other oxidized squalene derivatives like squalene-2,3-epoxide (2,3-oxidosqualene) .
This compound is naturally occurring and has been identified in plant systems, such as Glycyrrhiza glabra (licorice), where it serves as a precursor in the biosynthetic pathways of soyasaponins, betulinic acid, and glycyrrhizin . Industrially, this compound is available in high purity (≥98%) and is used in life sciences research, particularly in studies involving lipid metabolism and enzyme inhibition . It is stored at -20°C (stable for 3–6 months) or -80°C (stable for 12 months) to prevent degradation .
Vorbereitungsmethoden
Enzymatic and Biosynthetic Approaches
Squalene Cyclase-Catalyzed Reactions
Squalene cyclase, a key enzyme in sterol biosynthesis, catalyzes the cyclization of squalene derivatives into polycyclic structures. Recent studies demonstrate its utility in producing squalene-2,3-diol through controlled oxidation and cyclization. For instance, enzymatic treatment of (3R)- and (3S)-2,3-squalene diols with squalene cyclase generates bicyclic compounds and epoxydammaranes in a ~3:2 ratio . This reaction proceeds via a 6/6/6/5-fused tetracyclic carbocation intermediate, where the stereochemistry of the hydroxyl group dictates product distribution . The rigidity of the enzyme’s reaction cavity enforces an all-chair conformation on the substrate, ensuring high stereochemical fidelity .
Microbial Engineering and Fermentation
Advances in metabolic engineering have enabled microbial overproduction of this compound. Engineered strains of Saccharomyces cerevisiae and Yarrowia lipolytica optimize squalene biosynthesis pathways by overexpressing squalene synthase (SQS) and 2,3-oxidosqualene cyclase (OSC) . For example, persimmon fruit studies reveal that OA and UA biosynthesis correlates with OSC activity, suggesting analogous pathways for diol production . Fermentation protocols typically involve:
-
Substrate feeding : Supplementing cultures with mevalonate pathway intermediates (e.g., farnesyl pyrophosphate) .
-
Oxidation : Enzymatic conversion of squalene to 2,3-oxidosqualene via squalene monooxygenase, followed by hydrolysis to the diol .
Chemical Synthesis Strategies
Catalytic Asymmetric Dihydroxylation
The Sharpless asymmetric dihydroxylation offers a stereoselective route to vicinal diols. Applied to squalene, this method targets specific double bonds for oxidation. For example, using osmium tetroxide (OsO₄) with a chiral ligand (e.g., (DHQ)₂PHAL) and N-methylmorpholine N-oxide (NMO) as a cooxidant, the 2,3-double bond undergoes dihydroxylation to yield this compound . Key parameters include:
-
Temperature : 0–25°C.
-
Solvent : tert-Butanol/water mixtures.
Table 1: Dihydroxylation Conditions and Outcomes
Reagent System | Solvent | Temperature | Yield (%) | ee (%) |
---|---|---|---|---|
OsO₄/(DHQ)₂PHAL/NMO | t-BuOH/H₂O | 0°C | 68 | 92 |
OsO₄/Quinuclidine | Acetone/H₂O | 25°C | 72 | 88 |
Epoxidation and Acid-Catalyzed Hydrolysis
Epoxidation of squalene’s 2,3-double bond followed by ring-opening provides an alternative route. Meta-chloroperbenzoic acid (mCPBA) in dichloromethane selectively epoxidizes squalene to 2,3-epoxysqualene, which is hydrolyzed under acidic conditions (e.g., HCl in THF/H₂O) to the diol. This method faces challenges in regioselectivity due to squalene’s six double bonds, but steric hindrance at the 2,3-position favors targeted epoxidation.
Extraction and Purification from Natural Sources
Supercritical Fluid Extraction (SFE)
SFE with carbon dioxide (SC-CO₂) isolates squalene from plant oils (e.g., amaranth, olive), which is subsequently oxidized to the diol. Optimal conditions include:
-
Pressure : 200–300 bar.
-
Temperature : 50–60°C.
-
Co-solvent : Ethanol (10–15%) enhances polarity and yield .
Post-extraction, molecular distillation at 180°C and 3 mtorr concentrates this compound ninefold with 67.8% recovery .
Table 2: SFE Parameters for Squalene Enrichment
Source | Pressure (bar) | Temperature (°C) | Co-solvent | Yield (g/100 g) |
---|---|---|---|---|
Amaranth oil | 200 | 50 | 10% EtOH | 0.31 |
Olive oil | 250 | 55 | 15% EtOH | 0.28 |
Alkali Refining and Vacuum Distillation
Crude plant oils undergo alkali refining to remove free fatty acids, followed by vacuum distillation to isolate this compound. Distillation at 180°C and 3 mtorr achieves 76% recovery, though residual fatty acids may necessitate additional purification steps (e.g., column chromatography) .
Industrial-Scale Production Challenges
-
Enzymatic Methods : High enzyme costs and low volumetric productivity limit scalability .
-
Chemical Synthesis : Toxic reagents (e.g., OsO₄) and regioselectivity issues complicate large-scale use .
-
Extraction : Low squalene concentrations in natural sources (~0.1–0.3% in amaranth oil) necessitate energy-intensive processing .
Emerging Technologies
Analyse Chemischer Reaktionen
Types of Reactions: Squalene-2,3-diol undergoes various chemical reactions, including oxidation, reduction, and substitution .
Common Reagents and Conditions:
Oxidation: Common oxidizing agents include potassium permanganate and chromium trioxide.
Reduction: Reducing agents such as lithium aluminum hydride and sodium borohydride are used.
Substitution: Halogenation reactions can be carried out using reagents like bromine or chlorine.
Major Products: The major products formed from these reactions depend on the specific reagents and conditions used. For example, oxidation can yield squalene-2,3-dione, while reduction can produce squalane .
Wissenschaftliche Forschungsanwendungen
Chemical Properties and Biosynthesis
Squalene-2,3-diol is a triterpenoid compound that plays a crucial role in the biosynthesis of sterols and other bioactive compounds. Its structure allows it to participate in various biochemical pathways, including the synthesis of carotenoids and sterols. Recent studies have shown that squalene serves as a precursor for C30 carotenoid biosynthesis, expanding its functional repertoire beyond traditional associations with polycyclic triterpenes .
Pharmaceutical Applications
1. Antitumor Activity
this compound has been investigated for its potential anticancer properties. Research indicates that squalenoylated nanoparticles can target cancer cells effectively due to their ability to dissolve in low-density lipoproteins (LDL), which are often overexpressed in tumor tissues. This targeting mechanism enhances the delivery of therapeutic agents specifically to cancer cells, improving treatment efficacy .
2. Immunomodulatory Effects
Studies have highlighted the immunomodulatory effects of squalene derivatives. This compound has shown potential in modulating immune responses, which could be beneficial in treating autoimmune diseases and enhancing vaccine efficacy . For instance, its role as an adjuvant in vaccine formulations has been explored to improve immune responses against various pathogens.
3. Antioxidant Properties
The antioxidant capabilities of this compound contribute to its protective effects against oxidative stress-related diseases. It has been shown to mitigate oxidative damage in cellular models, suggesting its potential use as a therapeutic agent in conditions characterized by oxidative stress .
Cosmetic Applications
1. Skin Health
this compound is widely used in cosmetic formulations due to its moisturizing properties and ability to enhance skin barrier function. Its emollient characteristics make it an effective ingredient for hydrating skin products . Clinical studies have demonstrated that formulations containing squalene derivatives can improve skin hydration and elasticity without causing irritation.
2. Anti-Aging Effects
The compound's antioxidant properties also lend themselves to anti-aging applications. By reducing oxidative stress and promoting skin cell regeneration, this compound can help mitigate signs of aging such as wrinkles and fine lines .
Case Studies
Wirkmechanismus
Squalene-2,3-diol exerts its effects through various molecular targets and pathways:
Antioxidant Activity: It scavenges free radicals, thereby protecting cells from oxidative stress.
Anti-inflammatory Effects: It inhibits the production of pro-inflammatory cytokines, reducing inflammation.
Antibacterial Properties: It disrupts bacterial cell membranes, leading to cell lysis.
Vergleich Mit ähnlichen Verbindungen
Structural and Functional Comparison
Table 1: Key Structural and Functional Differences
Squalene-2,3-epoxide
- Role in Biosynthesis: Squalene-2,3-epoxide is a critical intermediate in the cholesterol biosynthesis pathway. SQLE (squalene epoxidase) catalyzes the epoxidation of squalene to form this compound, which is further cyclized to lanosterol in vertebrates or cycloartenol in plants .
- Enzymatic Processing: Unlike this compound, squalene-2,3-epoxide is directly involved in cyclization reactions. In human granulocytes, squalene-2,3-epoxide is converted to lanosterol but cannot proceed to cholesterol due to deficient downstream oxidase activity .
- Therapeutic Relevance : Inhibitors of SQLE (e.g., terbinafine) are used as antifungal agents, highlighting the importance of squalene-2,3-epoxide in pathogen lipid metabolism .
2,3:22,23-Diepoxysqualene
- Formation and Fate : SQLE can introduce a second epoxide group into squalene-2,3-epoxide to form 2,3:22,23-diepoxysqualene. This compound is metabolized to 24(S),25-epoxycholesterol (24S,25-EC), a regulatory oxysterol, bypassing cholesterol synthesis .
- Metabolic Flux: In human adipose tissue, downstream products of squalene-2,3-epoxide (e.g., 24S,25-EC) are rapidly converted, making their accumulation negligible under normal conditions .
Malabaricanediol (3)
- Synthetic Origin: Malabaricanediol is a cyclized triterpenoid derived from squalene-2,3-epoxide via non-enzymatic reactions using catalysts like stannic chloride or picric acid .
- Structural Divergence : Unlike this compound, malabaricanediol features a fused cyclic structure, limiting its role in linear sterol biosynthesis .
Enzymatic and Metabolic Differences
- In contrast, squalene-2,3-epoxide is the primary SQLE product and a rate-limiting intermediate in sterol synthesis .
- Downstream Processing: this compound is metabolized in plants to triterpenoids (e.g., β-amyrin), while squalene-2,3-epoxide is funneled into both sterols (cholesterol, phytosterols) and oxysterols .
Research Findings and Implications
- Inhibition Studies: Terbinafine, an SQLE inhibitor, causes squalene accumulation and ergosterol depletion in Trypanosoma cruzi, validating squalene-2,3-epoxide as a drug target .
- Metabolic Deficiencies: Human granulocytes lack enzymes to process lanosterol (derived from squalene-2,3-epoxide) into cholesterol, underscoring tissue-specific differences in sterol metabolism .
- Plant Biosynthesis : In Glycyrrhiza glabra, this compound is a branch-point metabolite, directing carbon flux toward soyasaponins or betulinic acid .
Biologische Aktivität
Squalene-2,3-diol is a diol derivative of squalene, a natural triterpene hydrocarbon. This compound has garnered attention due to its potential biological activities, particularly in the realms of cancer treatment, antibacterial properties, and its role in cholesterol biosynthesis. This article delves into the biological activity of this compound, summarizing key research findings, mechanisms of action, and potential applications.
This compound (C30H52O2) is characterized by hydroxyl groups at the 2 and 3 positions on the squalene backbone. This structural feature is crucial for its biological activity as it influences reactivity and interaction with biological targets.
Mechanism of Action:
- Biosynthesis Role: this compound serves as an intermediate in the enzymatic conversion of squalene to lanosterol and subsequently to cholesterol. It plays a pivotal role in the sterol biosynthetic pathway, which is essential for maintaining cellular membrane integrity and fluidity.
- Cellular Effects: The compound influences cell signaling pathways and gene expression, impacting cellular metabolism and function. It may also interact with various biomolecules through enzyme inhibition or activation.
Anticancer Properties
Research indicates that this compound may possess anticancer properties. Preliminary studies have shown that it can induce apoptosis (programmed cell death) in certain cancer cell lines. The precise mechanisms remain largely unexplored but may involve:
- Induction of oxidative stress leading to cell death.
- Modulation of signaling pathways associated with cell proliferation and survival .
A notable study highlighted that squalene derivatives could inhibit tumor growth in animal models when administered before or during carcinogen exposure. Squalene's protective effects against various carcinogens were documented, suggesting a potential chemopreventive role .
Antibacterial Activity
This compound has demonstrated effectiveness against antibiotic-resistant bacteria such as Pseudomonas aeruginosa, which poses significant challenges in clinical settings. Its antibacterial properties suggest that it could be utilized as a natural antimicrobial agent .
Pharmacokinetics and Safety
While the pharmacokinetics of this compound specifically require further investigation, related studies on squalene-containing compounds indicate that these substances can be rapidly metabolized and cleared from the body following administration. Understanding the pharmacokinetic profile is essential for evaluating the safety and efficacy of this compound in therapeutic applications.
Applications
This compound's diverse biological activities open avenues for various applications:
- Pharmaceuticals: Potential use as an anticancer agent or adjunct therapy in cancer treatment.
- Antimicrobial formulations: Development of new antibacterial agents targeting resistant strains.
- Cosmetics: Utilization in skincare products for its moisturizing and antioxidant properties .
Comparative Analysis Table
Compound | Structure Features | Biological Role | Unique Aspects |
---|---|---|---|
Squalene | Hydrocarbon with multiple double bonds | Precursor for sterols | Lacks hydroxyl groups |
This compound | Hydroxyl groups at C2 and C3 | Intermediate in cholesterol biosynthesis | Influences reactivity due to hydroxyl positioning |
Squalene-2,3-oxide | Oxidized form of squalene | Intermediate in sterol biosynthesis | Contains an epoxide group |
Lanosterol | Cyclization product of squalene | Key sterol in cholesterol synthesis | Contains multiple rings |
Cholesterol | Steroid structure | Essential for cell membranes | Contains a hydroxyl group at C3 |
Q & A
Basic Research Questions
Q. What is the metabolic role of squalene-2,3-diol in sterol biosynthesis, and how can its intermediates be experimentally tracked?
this compound is a key intermediate in sterol biosynthesis, formed via the epoxidation of squalene by squalene monooxygenase (EC 1.14.14.17). This step precedes cyclization into lanosterol or cycloartenol, depending on the organism . To track intermediates, researchers employ GC-MS with selected-ion monitoring (SIM) to separate and quantify squalene derivatives (e.g., squalene-2,3-epoxide, lanosterol) in biological matrices like T. cruzi. Optimization of temperature gradients and ion fragmentation patterns is critical for resolution .
Q. What analytical methods are recommended for quantifying this compound and its derivatives in complex biological samples?
Gas chromatography-mass spectrometry (GC-MS) is the gold standard, particularly for simultaneous detection of neutral lipids. A validated protocol involves:
- Derivatization (e.g., silylation) to enhance volatility.
- SIM mode targeting ions such as m/z 69 (squalene) and m/z 131 (squalene-2,3-epoxide).
- Chromatographic separation using a 5% phenyl-methylpolysiloxane column with a 10°C/min temperature ramp . Internal standards (e.g., deuterated cholesterol) improve accuracy in lipid-rich matrices .
Q. How can researchers ensure the stability of this compound during storage and experimental workflows?
Stability protocols include:
- Storing this compound powder at -80°C in inert atmospheres to prevent oxidation.
- Preparing fresh stock solutions in anhydrous DMSO to avoid hydrolysis.
- Validating storage conditions via periodic LC-MS purity checks .
Advanced Research Questions
Q. What experimental strategies resolve contradictions in enzymatic vs. non-enzymatic cyclization pathways of squalene-2,3-epoxide?
Contradictions arise from studies showing both enzymatic (lanosterol synthase) and non-enzymatic cyclization of squalene-2,3-epoxide. To differentiate:
- Use isotopic labeling (e.g., ¹⁴C-squalene) to trace enzymatic turnover in liver microsomes vs. abiotic conditions .
- Apply enzyme inhibitors (e.g., terbinafine for squalene epoxidase) to isolate non-enzymatic contributions .
- Compare product stereochemistry via NMR; enzymatic cyclization yields specific stereoisomers (e.g., lanosterol), while non-enzymatic routes produce racemic mixtures .
Q. How can researchers develop a robust GC-MS method for quantifying this compound in understudied biological matrices (e.g., protozoan parasites)?
Key steps include:
- Matrix-specific optimization: Adjust lipid extraction protocols (e.g., Folch method) to minimize co-eluting contaminants.
- Validation parameters: Assess linearity (0.1–50 µg/mL), limit of detection (LOD < 0.05 µg/mL), and recovery rates (>85%) using spiked samples .
- Cross-validate with orthogonal techniques like LC-APCI-MS to confirm specificity .
Q. What role does this compound play in plant vs. animal sterol biosynthesis, and how can these pathways be distinguished experimentally?
In plants, squalene-2,3-epoxide is cyclized to cycloartenol by cycloartenol synthase (EC 5.4.99.8), while animals produce lanosterol via lanosterol synthase. To differentiate:
- Use species-specific enzyme inhibitors (e.g., AMO 1618 for plant cycloartenol synthase).
- Perform tracer experiments with ¹³C-labeled precursors and analyze products via high-resolution MS .
Q. How can researchers address reproducibility challenges in this compound-related studies, particularly in enzyme activity assays?
Mitigation strategies include:
- Standardizing enzyme sources (e.g., recombinant squalene monooxygenase expressed in E. coli).
- Pre-treating substrates with antioxidants (e.g., BHT) to prevent auto-oxidation during assays.
- Reporting detailed kinetic parameters (e.g., Kₘ, Vₘₐₓ) and normalizing data to protein concentration .
Q. Methodological Notes
- Data Analysis : Use tools like Skyline or XCMS for untargeted lipidomics to identify squalene derivatives .
- Ethical Reporting : Follow guidelines from Cochrane Reviews for systematic data synthesis, including bias reduction through multi-source validation .
- Experimental Design : Incorporate negative controls (e.g., heat-inactivated enzymes) and triplicate runs to ensure statistical rigor .
Eigenschaften
IUPAC Name |
(6E,10E,14E,18E)-2,6,10,15,19,23-hexamethyltetracosa-6,10,14,18,22-pentaene-2,3-diol | |
---|---|---|
Source | PubChem | |
URL | https://pubchem.ncbi.nlm.nih.gov | |
Description | Data deposited in or computed by PubChem | |
InChI |
InChI=1S/C30H52O2/c1-24(2)14-11-17-27(5)20-12-18-25(3)15-9-10-16-26(4)19-13-21-28(6)22-23-29(31)30(7,8)32/h14-16,20-21,29,31-32H,9-13,17-19,22-23H2,1-8H3/b25-15+,26-16+,27-20+,28-21+ | |
Source | PubChem | |
URL | https://pubchem.ncbi.nlm.nih.gov | |
Description | Data deposited in or computed by PubChem | |
InChI Key |
GRPNWQFOKYUABH-BANQPHDMSA-N | |
Source | PubChem | |
URL | https://pubchem.ncbi.nlm.nih.gov | |
Description | Data deposited in or computed by PubChem | |
Canonical SMILES |
CC(=CCCC(=CCCC(=CCCC=C(C)CCC=C(C)CCC(C(C)(C)O)O)C)C)C | |
Source | PubChem | |
URL | https://pubchem.ncbi.nlm.nih.gov | |
Description | Data deposited in or computed by PubChem | |
Isomeric SMILES |
CC(=CCC/C(=C/CC/C(=C/CC/C=C(\C)/CC/C=C(\C)/CCC(C(C)(C)O)O)/C)/C)C | |
Source | PubChem | |
URL | https://pubchem.ncbi.nlm.nih.gov | |
Description | Data deposited in or computed by PubChem | |
Molecular Formula |
C30H52O2 | |
Source | PubChem | |
URL | https://pubchem.ncbi.nlm.nih.gov | |
Description | Data deposited in or computed by PubChem | |
Molecular Weight |
444.7 g/mol | |
Source | PubChem | |
URL | https://pubchem.ncbi.nlm.nih.gov | |
Description | Data deposited in or computed by PubChem | |
Haftungsausschluss und Informationen zu In-Vitro-Forschungsprodukten
Bitte beachten Sie, dass alle Artikel und Produktinformationen, die auf BenchChem präsentiert werden, ausschließlich zu Informationszwecken bestimmt sind. Die auf BenchChem zum Kauf angebotenen Produkte sind speziell für In-vitro-Studien konzipiert, die außerhalb lebender Organismen durchgeführt werden. In-vitro-Studien, abgeleitet von dem lateinischen Begriff "in Glas", beinhalten Experimente, die in kontrollierten Laborumgebungen unter Verwendung von Zellen oder Geweben durchgeführt werden. Es ist wichtig zu beachten, dass diese Produkte nicht als Arzneimittel oder Medikamente eingestuft sind und keine Zulassung der FDA für die Vorbeugung, Behandlung oder Heilung von medizinischen Zuständen, Beschwerden oder Krankheiten erhalten haben. Wir müssen betonen, dass jede Form der körperlichen Einführung dieser Produkte in Menschen oder Tiere gesetzlich strikt untersagt ist. Es ist unerlässlich, sich an diese Richtlinien zu halten, um die Einhaltung rechtlicher und ethischer Standards in Forschung und Experiment zu gewährleisten.