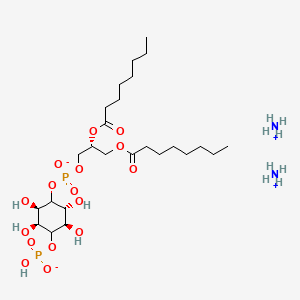
PtdIns-(4)-P1 (1,2-dioctanoyl) (ammonium salt)
- Klicken Sie auf QUICK INQUIRY, um ein Angebot von unserem Expertenteam zu erhalten.
- Mit qualitativ hochwertigen Produkten zu einem WETTBEWERBSFÄHIGEN Preis können Sie sich mehr auf Ihre Forschung konzentrieren.
Übersicht
Beschreibung
Inositol phospholipid species are membrane-bound signaling molecules that have been implicated in almost all aspects of cellular physiology, including cellular growth, metabolism, proliferation, and survival.
08:0 PIP is a phosphoinositol phosphate (PIP). Among prokaryotes, PI is synthesized by mycobacteria and few other bacterial genera.
The phosphatidylinositol (PtdIns) phosphates represent a small percentage of total membrane phospholipids. However, they play a critical role in the generation and transmission of cellular signals. PtdIns-(4)-P1 (1,2-dioctanoyl) is a synthetic analog of natural phosphatidylinositol (PtdIns) featuring C8:0 fatty acids at the sn-1 and sn-2 positions. The compound contains the same inositol and diacylglycerol (DAG) stereochemistry as the natural compound. PtdIns-(4)-P1 can be phosphorylated to di- (PtdIns-P2; PIP2) and triphosphates (PtdIns-P3; PIP3). Hydrolysis of PtdIns-(4,5)-P2 by phosphoinositide (PI)-specific phospholipase C generates inositol triphosphate (IP3) and DAG which are key second messengers in an intricate biochemical signal transduction cascade.
Wirkmechanismus
Target of Action
PtdIns-(4)-P1 (1,2-dioctanoyl) (ammonium salt), also known as diazanium;[(2S,3R,5R,6R)-4-[[(2R)-2,3-di(octanoyloxy)propoxy]-oxidophosphoryl]oxy-2,3,5,6-tetrahydroxycyclohexyl] hydrogen phosphate, is a synthetic analog of natural phosphatidylinositol (PtdIns) containing C8:0 fatty acids at the sn - 1 and sn - 2 positions . The primary targets of this compound are the cellular signals that play a critical role in the generation and transmission .
Mode of Action
The compound features the same inositol and diacyl glycerol (DAG) stereochemistry as that of the natural compound . It interacts with its targets by being phosphorylated to mono- (PtdIns- P; PIP), di- (PtdIns- P 2; PIP 2 ), and triphosphates (PtdIns- P 3; PIP 3 ) .
Biochemical Pathways
The biochemical pathways affected by this compound involve the hydrolysis of PtdIns- (4,5)- P 2 by phosphoinositide (PI)- specific phospholipase C, which generates inositol triphosphate (IP 3) and DAG . These are key second messengers in an intricate biochemical signal transduction cascade .
Pharmacokinetics
The short fatty acid chains of this analog, compared to naturally-occurring PtdIns, give it different physical properties including high solubility in aqueous media . This property can impact the compound’s ADME (Absorption, Distribution, Metabolism, and Excretion) properties and their impact on bioavailability.
Result of Action
The result of the compound’s action is the generation and transmission of cellular signals . The DAG and IP 3 produced by the hydrolysis of PtdIns- (4,5)- P 2 are part of a complex biochemical and signal transduction cascade .
Biochemische Analyse
Biochemical Properties
PtdIns-(4)-P1 (1,2-dioctanoyl) (ammonium salt) is involved in intricate biochemical signal transduction cascades . It interacts with various enzymes and proteins, playing a crucial role in the generation and transmission of cellular signals . The compound features the same inositol and diacyl glycerol (DAG) stereochemistry as that of the natural compound .
Cellular Effects
The effects of PtdIns-(4)-P1 (1,2-dioctanoyl) (ammonium salt) on various types of cells and cellular processes are significant. It influences cell function, including impacts on cell signaling pathways, gene expression, and cellular metabolism .
Molecular Mechanism
PtdIns-(4)-P1 (1,2-dioctanoyl) (ammonium salt) exerts its effects at the molecular level through various mechanisms. It can bind to important proteins such as Group IV cPLA 2 and PLCδ1, enhancing their adherence to the membrane and increasing the rate of substrate hydrolysis . It can also be further phosphorylated to give triphosphates such as PtdIns- (3,4,5)- P 3 .
Metabolic Pathways
PtdIns-(4)-P1 (1,2-dioctanoyl) (ammonium salt) is involved in several metabolic pathways. It interacts with various enzymes or cofactors, and can also affect metabolic flux or metabolite levels .
Eigenschaften
IUPAC Name |
diazanium;[(2R,3R,5R,6S)-4-[[(2R)-2,3-di(octanoyloxy)propoxy]-oxidophosphoryl]oxy-2,3,5,6-tetrahydroxycyclohexyl] hydrogen phosphate |
Source
|
---|---|---|
Source | PubChem | |
URL | https://pubchem.ncbi.nlm.nih.gov | |
Description | Data deposited in or computed by PubChem | |
InChI |
InChI=1S/C25H48O16P2.2H3N/c1-3-5-7-9-11-13-18(26)37-15-17(39-19(27)14-12-10-8-6-4-2)16-38-43(35,36)41-25-22(30)20(28)24(21(29)23(25)31)40-42(32,33)34;;/h17,20-25,28-31H,3-16H2,1-2H3,(H,35,36)(H2,32,33,34);2*1H3/t17-,20-,21+,22-,23-,24?,25?;;/m1../s1 |
Source
|
Source | PubChem | |
URL | https://pubchem.ncbi.nlm.nih.gov | |
Description | Data deposited in or computed by PubChem | |
InChI Key |
GRYUDEZZGXKOJR-XMXDBXOESA-N |
Source
|
Source | PubChem | |
URL | https://pubchem.ncbi.nlm.nih.gov | |
Description | Data deposited in or computed by PubChem | |
Canonical SMILES |
CCCCCCCC(=O)OCC(COP(=O)([O-])OC1C(C(C(C(C1O)O)OP(=O)(O)[O-])O)O)OC(=O)CCCCCCC.[NH4+].[NH4+] |
Source
|
Source | PubChem | |
URL | https://pubchem.ncbi.nlm.nih.gov | |
Description | Data deposited in or computed by PubChem | |
Isomeric SMILES |
CCCCCCCC(=O)OC[C@H](COP(=O)([O-])OC1[C@@H]([C@H](C([C@H]([C@H]1O)O)OP(=O)(O)[O-])O)O)OC(=O)CCCCCCC.[NH4+].[NH4+] |
Source
|
Source | PubChem | |
URL | https://pubchem.ncbi.nlm.nih.gov | |
Description | Data deposited in or computed by PubChem | |
Molecular Formula |
C25H54N2O16P2 |
Source
|
Source | PubChem | |
URL | https://pubchem.ncbi.nlm.nih.gov | |
Description | Data deposited in or computed by PubChem | |
Molecular Weight |
700.6 g/mol |
Source
|
Source | PubChem | |
URL | https://pubchem.ncbi.nlm.nih.gov | |
Description | Data deposited in or computed by PubChem | |
Haftungsausschluss und Informationen zu In-Vitro-Forschungsprodukten
Bitte beachten Sie, dass alle Artikel und Produktinformationen, die auf BenchChem präsentiert werden, ausschließlich zu Informationszwecken bestimmt sind. Die auf BenchChem zum Kauf angebotenen Produkte sind speziell für In-vitro-Studien konzipiert, die außerhalb lebender Organismen durchgeführt werden. In-vitro-Studien, abgeleitet von dem lateinischen Begriff "in Glas", beinhalten Experimente, die in kontrollierten Laborumgebungen unter Verwendung von Zellen oder Geweben durchgeführt werden. Es ist wichtig zu beachten, dass diese Produkte nicht als Arzneimittel oder Medikamente eingestuft sind und keine Zulassung der FDA für die Vorbeugung, Behandlung oder Heilung von medizinischen Zuständen, Beschwerden oder Krankheiten erhalten haben. Wir müssen betonen, dass jede Form der körperlichen Einführung dieser Produkte in Menschen oder Tiere gesetzlich strikt untersagt ist. Es ist unerlässlich, sich an diese Richtlinien zu halten, um die Einhaltung rechtlicher und ethischer Standards in Forschung und Experiment zu gewährleisten.