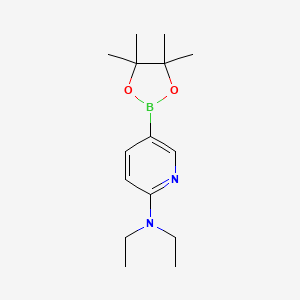
N,N-diethyl-5-(4,4,5,5-tetramethyl-1,3,2-dioxaborolan-2-yl)pyridin-2-amine
- Klicken Sie auf QUICK INQUIRY, um ein Angebot von unserem Expertenteam zu erhalten.
- Mit qualitativ hochwertigen Produkten zu einem WETTBEWERBSFÄHIGEN Preis können Sie sich mehr auf Ihre Forschung konzentrieren.
Übersicht
Beschreibung
N,N-diethyl-5-(4,4,5,5-tetramethyl-1,3,2-dioxaborolan-2-yl)pyridin-2-amine is an organic compound that features a pyridine ring substituted with a diethylamino group and a dioxaborolane moiety
Vorbereitungsmethoden
Synthetic Routes and Reaction Conditions
The synthesis of N,N-diethyl-5-(4,4,5,5-tetramethyl-1,3,2-dioxaborolan-2-yl)pyridin-2-amine typically involves the borylation of a pyridine derivative. One common method is the palladium-catalyzed borylation of a halogenated pyridine with bis(pinacolato)diboron under inert conditions. The reaction is usually carried out in the presence of a base such as potassium carbonate and a ligand like triphenylphosphine .
Industrial Production Methods
Industrial production of this compound may involve similar synthetic routes but on a larger scale. The use of continuous flow reactors and automated systems can enhance the efficiency and yield of the process. Additionally, purification steps such as recrystallization or chromatography are employed to obtain the compound in high purity.
Analyse Chemischer Reaktionen
Types of Reactions
N,N-diethyl-5-(4,4,5,5-tetramethyl-1,3,2-dioxaborolan-2-yl)pyridin-2-amine can undergo various chemical reactions, including:
Oxidation: The compound can be oxidized to form corresponding N-oxides.
Reduction: Reduction reactions can convert the pyridine ring to a piperidine ring.
Substitution: The compound can participate in nucleophilic substitution reactions, particularly at the pyridine ring.
Common Reagents and Conditions
Oxidation: Common oxidizing agents include hydrogen peroxide and m-chloroperbenzoic acid.
Reduction: Reducing agents such as lithium aluminum hydride or sodium borohydride are used.
Substitution: Nucleophiles like amines or thiols can be used under basic conditions.
Major Products
The major products formed from these reactions depend on the specific conditions and reagents used. For example, oxidation may yield N-oxides, while reduction can produce piperidine derivatives.
Wissenschaftliche Forschungsanwendungen
N,N-diethyl-5-(4,4,5,5-tetramethyl-1,3,2-dioxaborolan-2-yl)pyridin-2-amine has several applications in scientific research:
Biology: The compound can be used in the development of bioactive molecules and as a probe in biological studies.
Industry: It is used in the production of advanced materials, such as polymers and electronic components.
Wirkmechanismus
The mechanism by which N,N-diethyl-5-(4,4,5,5-tetramethyl-1,3,2-dioxaborolan-2-yl)pyridin-2-amine exerts its effects involves interactions with molecular targets such as enzymes or receptors. The dioxaborolane moiety can form reversible covalent bonds with nucleophilic sites, while the pyridine ring can engage in π-π interactions with aromatic residues. These interactions can modulate the activity of biological pathways and processes .
Vergleich Mit ähnlichen Verbindungen
Similar Compounds
N,N-Dimethyl-4-(4,4,5,5-tetramethyl-1,3,2-dioxaborolan-2-yl)aniline: This compound features a similar dioxaborolane moiety but with a dimethylamino group instead of a diethylamino group.
4-(4,4,5,5-Tetramethyl-1,3,2-dioxaborolan-2-yl)benzaldehyde: This compound has a benzaldehyde group instead of a pyridine ring.
Uniqueness
N,N-diethyl-5-(4,4,5,5-tetramethyl-1,3,2-dioxaborolan-2-yl)pyridin-2-amine is unique due to its combination of a pyridine ring and a diethylamino group, which can provide distinct electronic and steric properties compared to similar compounds. This uniqueness makes it valuable in specific synthetic applications and research studies.
Biologische Aktivität
N,N-Diethyl-5-(4,4,5,5-tetramethyl-1,3,2-dioxaborolan-2-yl)pyridin-2-amine (CAS No. 1311165-58-8) is a compound of interest due to its potential biological activities. This article reviews the biological activity of this compound based on available literature and research findings.
- Molecular Formula: C15H25BN2O2
- Molecular Weight: 304.19 g/mol
- Structure: The compound features a pyridine ring substituted with a dioxaborolane moiety, which is known for its role in drug design and development.
Biological Activity Overview
The biological activity of this compound has been explored in various studies focusing on its pharmacological potential. Key areas of investigation include:
-
Anticancer Activity
- Studies have indicated that compounds with similar structures exhibit significant anticancer properties. For instance, pyridine derivatives have shown potent inhibitory effects on various cancer cell lines.
- In vitro assays demonstrated that related compounds can inhibit cell proliferation in cancer models with IC50 values often in the nanomolar range .
-
Mechanism of Action
- The mechanism through which these compounds exert their effects often involves the inhibition of specific enzymes or pathways critical for cancer cell survival and proliferation. For example, some derivatives have been shown to inhibit matrix metalloproteinases (MMPs), which are involved in tumor invasion and metastasis .
- Additionally, the presence of the dioxaborolane group may enhance the compound's ability to interact with biological targets due to its unique electronic properties .
-
Toxicity and Safety Profile
- Preliminary toxicity assessments indicate that while some derivatives exhibit toxicity at high concentrations (e.g., H301: Toxic if swallowed), others demonstrate a favorable safety profile in vivo .
- A study involving oral administration in mice showed no significant adverse effects at therapeutic doses, suggesting potential for further development as a therapeutic agent .
Case Studies
Several case studies have been documented regarding the biological activity of similar compounds:
- Pyridine Derivatives in Cancer Therapy
- In Vivo Efficacy
Data Summary Table
Property | Value |
---|---|
Molecular Formula | C15H25BN2O2 |
Molecular Weight | 304.19 g/mol |
CAS Number | 1311165-58-8 |
IC50 (Cancer Cell Lines) | 0.126 μM (example) |
Toxicity Classification | H301 (Toxic if swallowed) |
Safety Profile | Favorable at therapeutic doses |
Eigenschaften
IUPAC Name |
N,N-diethyl-5-(4,4,5,5-tetramethyl-1,3,2-dioxaborolan-2-yl)pyridin-2-amine |
Source
|
---|---|---|
Source | PubChem | |
URL | https://pubchem.ncbi.nlm.nih.gov | |
Description | Data deposited in or computed by PubChem | |
InChI |
InChI=1S/C15H25BN2O2/c1-7-18(8-2)13-10-9-12(11-17-13)16-19-14(3,4)15(5,6)20-16/h9-11H,7-8H2,1-6H3 |
Source
|
Source | PubChem | |
URL | https://pubchem.ncbi.nlm.nih.gov | |
Description | Data deposited in or computed by PubChem | |
InChI Key |
XNHSDPDZFBPNOF-UHFFFAOYSA-N |
Source
|
Source | PubChem | |
URL | https://pubchem.ncbi.nlm.nih.gov | |
Description | Data deposited in or computed by PubChem | |
Canonical SMILES |
B1(OC(C(O1)(C)C)(C)C)C2=CN=C(C=C2)N(CC)CC |
Source
|
Source | PubChem | |
URL | https://pubchem.ncbi.nlm.nih.gov | |
Description | Data deposited in or computed by PubChem | |
Molecular Formula |
C15H25BN2O2 |
Source
|
Source | PubChem | |
URL | https://pubchem.ncbi.nlm.nih.gov | |
Description | Data deposited in or computed by PubChem | |
Molecular Weight |
276.18 g/mol |
Source
|
Source | PubChem | |
URL | https://pubchem.ncbi.nlm.nih.gov | |
Description | Data deposited in or computed by PubChem | |
Haftungsausschluss und Informationen zu In-Vitro-Forschungsprodukten
Bitte beachten Sie, dass alle Artikel und Produktinformationen, die auf BenchChem präsentiert werden, ausschließlich zu Informationszwecken bestimmt sind. Die auf BenchChem zum Kauf angebotenen Produkte sind speziell für In-vitro-Studien konzipiert, die außerhalb lebender Organismen durchgeführt werden. In-vitro-Studien, abgeleitet von dem lateinischen Begriff "in Glas", beinhalten Experimente, die in kontrollierten Laborumgebungen unter Verwendung von Zellen oder Geweben durchgeführt werden. Es ist wichtig zu beachten, dass diese Produkte nicht als Arzneimittel oder Medikamente eingestuft sind und keine Zulassung der FDA für die Vorbeugung, Behandlung oder Heilung von medizinischen Zuständen, Beschwerden oder Krankheiten erhalten haben. Wir müssen betonen, dass jede Form der körperlichen Einführung dieser Produkte in Menschen oder Tiere gesetzlich strikt untersagt ist. Es ist unerlässlich, sich an diese Richtlinien zu halten, um die Einhaltung rechtlicher und ethischer Standards in Forschung und Experiment zu gewährleisten.