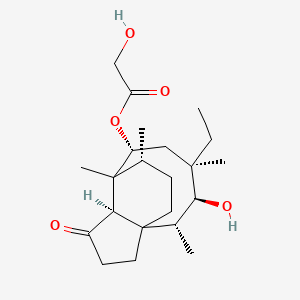
Dihydropleuromutilin
Übersicht
Beschreibung
Dihydropleuromutilin ist ein semisynthetisches Antibiotikum, das von Pleuromutilin abgeleitet ist, einem Naturstoff, der aus dem Pilz Clitopilus passeckerianus isoliert wurde. Diese Verbindung zeigt eine starke antibakterielle Aktivität, insbesondere gegen grampositive Bakterien, was sie zu einem wertvollen Gut bei der Entwicklung neuer Antibiotika macht .
Wirkmechanismus
Target of Action
Dihydropleuromutilin, a semi-synthetic derivative of pleuromutilin , primarily targets the peptidyl transferase center (PTC) of the bacterial ribosome . The PTC is responsible for catalyzing the formation of peptide bonds during protein synthesis .
Mode of Action
This compound inhibits bacterial protein synthesis by binding to the PTC of the ribosome . The interaction involves the binding of the C14 extension and the tricyclic core of the compound to the P and A sites of the PTC, respectively . This binding disrupts the protein synthesis process, leading to the inhibition of bacterial growth .
Biochemical Pathways
The primary biochemical pathway affected by this compound is the protein synthesis pathway in bacteria . By binding to the PTC of the ribosome, this compound prevents the formation of peptide bonds, thereby inhibiting the synthesis of proteins essential for bacterial survival and growth .
Pharmacokinetics
It is known that the compound exhibits antimicrobial activity against various bacteria, includingS. aureus, M. hominis, M. gallisepticum, and M. hyorhinis . More research is needed to fully understand the ADME properties of this compound and their impact on its bioavailability.
Result of Action
The result of this compound’s action is the inhibition of bacterial growth . By disrupting protein synthesis, this compound prevents bacteria from producing essential proteins, which ultimately leads to the inhibition of bacterial growth .
Action Environment
The action of this compound is influenced by various environmental factors. For instance, mutations in 23S rRNA, 50S ribosomal subunit proteins rplC and rplD, and ATP-binding cassette (ABC)-F transporter proteins can affect the activity of pleuromutilin antibiotics . .
Biochemische Analyse
Biochemical Properties
Dihydropleuromutilin interacts with the peptidyl transferase center (PTC) of the bacterial ribosome . This interaction involves the binding of the C14 extension and the tricyclic core to the P and A sites of the PTC, respectively . This interaction inhibits bacterial protein synthesis, making this compound an effective antibiotic .
Cellular Effects
The primary cellular effect of this compound is the inhibition of bacterial protein synthesis . By binding to the PTC of the bacterial ribosome, this compound prevents the formation of peptide bonds, thereby halting the production of proteins within the bacterial cell . This disruption in protein synthesis can lead to cell death, thereby exerting the antibiotic effect of this compound .
Molecular Mechanism
This compound exerts its effects at the molecular level through its interaction with the bacterial ribosome . Specifically, it binds to the PTC of the ribosome, preventing the formation of peptide bonds and thus inhibiting protein synthesis . This mechanism of action is what gives this compound its antibiotic properties .
Metabolic Pathways
The major metabolic pathway that deactivates pleuromutilin, the parent compound of this compound, involves cytochrome P450 oxidation at C2 and C8
Vorbereitungsmethoden
Synthetische Routen und Reaktionsbedingungen
Die Synthese von Dihydropleuromutilin beinhaltet die Hydrierung von Pleuromutilin. Dieser Prozess verwendet typischerweise einen Palladium-auf-Kohle-Katalysator unter Wasserstoffgas bei Raumtemperatur. Die Reaktionsbedingungen werden sorgfältig kontrolliert, um die selektive Reduktion der Doppelbindung in Pleuromutilin sicherzustellen, was zur Bildung von this compound führt .
Industrielle Produktionsverfahren
Die industrielle Produktion von this compound folgt ähnlichen Syntheserouten, jedoch in größerem Maßstab. Der Prozess beinhaltet die Verwendung von Hochdruckhydrierungsreaktoren und kontinuierlichen Fließsystemen, um die Effizienz und Ausbeute zu verbessern. Das Endprodukt wird durch Kristallisations- und Chromatographietechniken gereinigt, um die gewünschten Reinheitsgrade zu erreichen .
Analyse Chemischer Reaktionen
Arten von Reaktionen
Dihydropleuromutilin unterliegt verschiedenen chemischen Reaktionen, darunter:
Häufige Reagenzien und Bedingungen
Oxidation: Kaliumpermanganat in einem sauren Medium.
Reduktion: Natriumborhydrid in Methanol.
Substitution: Thionylchlorid in Dichlormethan.
Hauptprodukte, die gebildet werden
Oxidation: Hydroxylierte this compound-Derivate.
Reduktion: Reduzierte this compound-Derivate.
Substitution: Halogenierte this compound-Derivate.
Wissenschaftliche Forschungsanwendungen
Dihydropleuromutilin hat eine breite Palette von Anwendungen in der wissenschaftlichen Forschung:
Industrie: Wird bei der Entwicklung von Tierarzneimitteln und landwirtschaftlichen Antibiotika eingesetzt.
Wirkmechanismus
This compound übt seine antibakterielle Wirkung aus, indem es an das Peptidyltransferasezentrum des bakteriellen Ribosoms bindet. Diese Bindung hemmt die Bildung von Peptidbindungen während der Proteinsynthese und stoppt effektiv das bakterielle Wachstum und die Replikation . Die molekularen Zielstrukturen umfassen die 50S-Ribosomenuntereinheit, und die beteiligten Wege sind mit der Hemmung der Proteinsynthese verbunden .
Vergleich Mit ähnlichen Verbindungen
Ähnliche Verbindungen
Pleuromutilin: Die Stammverbindung, von der Dihydropleuromutilin abgeleitet ist.
Lefamulin: Ein semisynthetisches Pleuromutilin-Derivat mit verbesserter antibakterieller Aktivität.
Retapamulin: Ein weiteres Pleuromutilin-Derivat, das in topischen antibakteriellen Behandlungen verwendet wird.
Einzigartigkeit
This compound ist aufgrund seiner spezifischen Strukturmodifikationen einzigartig, die im Vergleich zu seiner Stammverbindung, Pleuromutilin, eine verbesserte Stabilität und antibakterielle Aktivität verleihen . Seine Fähigkeit, die bakterielle Proteinsynthese mit hoher Spezifität zu hemmen, macht es zu einer wertvollen Verbindung im Kampf gegen Antibiotika-resistente Bakterien .
Eigenschaften
IUPAC Name |
[(2R,3S,4R,6R,8R,14R)-4-ethyl-3-hydroxy-2,4,7,14-tetramethyl-9-oxo-6-tricyclo[5.4.3.01,8]tetradecanyl] 2-hydroxyacetate | |
---|---|---|
Source | PubChem | |
URL | https://pubchem.ncbi.nlm.nih.gov | |
Description | Data deposited in or computed by PubChem | |
InChI |
InChI=1S/C22H36O5/c1-6-20(4)11-16(27-17(25)12-23)21(5)13(2)7-9-22(14(3)19(20)26)10-8-15(24)18(21)22/h13-14,16,18-19,23,26H,6-12H2,1-5H3/t13-,14+,16-,18+,19+,20-,21?,22?/m1/s1 | |
Source | PubChem | |
URL | https://pubchem.ncbi.nlm.nih.gov | |
Description | Data deposited in or computed by PubChem | |
InChI Key |
RTOIPDPHQXGIRG-JKZASVEESA-N | |
Source | PubChem | |
URL | https://pubchem.ncbi.nlm.nih.gov | |
Description | Data deposited in or computed by PubChem | |
Canonical SMILES |
CCC1(CC(C2(C(CCC3(C2C(=O)CC3)C(C1O)C)C)C)OC(=O)CO)C | |
Source | PubChem | |
URL | https://pubchem.ncbi.nlm.nih.gov | |
Description | Data deposited in or computed by PubChem | |
Isomeric SMILES |
CC[C@@]1(C[C@H](C2([C@@H](CCC3([C@H]2C(=O)CC3)[C@H]([C@@H]1O)C)C)C)OC(=O)CO)C | |
Source | PubChem | |
URL | https://pubchem.ncbi.nlm.nih.gov | |
Description | Data deposited in or computed by PubChem | |
Molecular Formula |
C22H36O5 | |
Source | PubChem | |
URL | https://pubchem.ncbi.nlm.nih.gov | |
Description | Data deposited in or computed by PubChem | |
DSSTOX Substance ID |
DTXSID50676274 | |
Record name | (4R,5S,6R,8R,9aR,10R)-6-Ethyl-5-hydroxy-4,6,9,10-tetramethyl-1-oxodecahydro-3a,9-propanocyclopenta[8]annulen-8-yl hydroxyacetate | |
Source | EPA DSSTox | |
URL | https://comptox.epa.gov/dashboard/DTXSID50676274 | |
Description | DSSTox provides a high quality public chemistry resource for supporting improved predictive toxicology. | |
Molecular Weight |
380.5 g/mol | |
Source | PubChem | |
URL | https://pubchem.ncbi.nlm.nih.gov | |
Description | Data deposited in or computed by PubChem | |
CAS No. |
42302-24-9 | |
Record name | (4R,5S,6R,8R,9aR,10R)-6-Ethyl-5-hydroxy-4,6,9,10-tetramethyl-1-oxodecahydro-3a,9-propanocyclopenta[8]annulen-8-yl hydroxyacetate | |
Source | EPA DSSTox | |
URL | https://comptox.epa.gov/dashboard/DTXSID50676274 | |
Description | DSSTox provides a high quality public chemistry resource for supporting improved predictive toxicology. | |
Retrosynthesis Analysis
AI-Powered Synthesis Planning: Our tool employs the Template_relevance Pistachio, Template_relevance Bkms_metabolic, Template_relevance Pistachio_ringbreaker, Template_relevance Reaxys, Template_relevance Reaxys_biocatalysis model, leveraging a vast database of chemical reactions to predict feasible synthetic routes.
One-Step Synthesis Focus: Specifically designed for one-step synthesis, it provides concise and direct routes for your target compounds, streamlining the synthesis process.
Accurate Predictions: Utilizing the extensive PISTACHIO, BKMS_METABOLIC, PISTACHIO_RINGBREAKER, REAXYS, REAXYS_BIOCATALYSIS database, our tool offers high-accuracy predictions, reflecting the latest in chemical research and data.
Strategy Settings
Precursor scoring | Relevance Heuristic |
---|---|
Min. plausibility | 0.01 |
Model | Template_relevance |
Template Set | Pistachio/Bkms_metabolic/Pistachio_ringbreaker/Reaxys/Reaxys_biocatalysis |
Top-N result to add to graph | 6 |
Feasible Synthetic Routes
Haftungsausschluss und Informationen zu In-Vitro-Forschungsprodukten
Bitte beachten Sie, dass alle Artikel und Produktinformationen, die auf BenchChem präsentiert werden, ausschließlich zu Informationszwecken bestimmt sind. Die auf BenchChem zum Kauf angebotenen Produkte sind speziell für In-vitro-Studien konzipiert, die außerhalb lebender Organismen durchgeführt werden. In-vitro-Studien, abgeleitet von dem lateinischen Begriff "in Glas", beinhalten Experimente, die in kontrollierten Laborumgebungen unter Verwendung von Zellen oder Geweben durchgeführt werden. Es ist wichtig zu beachten, dass diese Produkte nicht als Arzneimittel oder Medikamente eingestuft sind und keine Zulassung der FDA für die Vorbeugung, Behandlung oder Heilung von medizinischen Zuständen, Beschwerden oder Krankheiten erhalten haben. Wir müssen betonen, dass jede Form der körperlichen Einführung dieser Produkte in Menschen oder Tiere gesetzlich strikt untersagt ist. Es ist unerlässlich, sich an diese Richtlinien zu halten, um die Einhaltung rechtlicher und ethischer Standards in Forschung und Experiment zu gewährleisten.