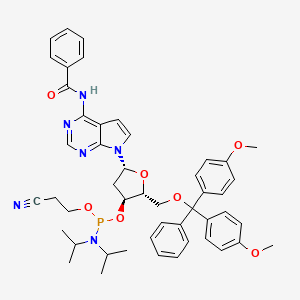
7-Deaza-DA cep
Übersicht
Beschreibung
7-Deaza-DA cep, also known as 7-deaza-2’-deoxyadenosine, is a modified nucleoside analog. It is structurally similar to deoxyadenosine but with a nitrogen atom replaced by a carbon atom at the seventh position of the purine ring. This modification imparts unique properties to the compound, making it valuable in various scientific research applications.
Vorbereitungsmethoden
Synthetic Routes and Reaction Conditions: The synthesis of 7-Deaza-DA cep typically involves the following steps:
Starting Material: The synthesis begins with commercially available 2’-deoxyadenosine.
Nitration: The 7-position of the purine ring is nitrated using a nitrating agent such as nitric acid.
Reduction: The nitro group is then reduced to an amino group using a reducing agent like hydrogen gas in the presence of a palladium catalyst.
Cyclization: The amino group is cyclized to form the 7-deaza structure using a cyclizing agent such as phosphorus oxychloride.
Industrial Production Methods: Industrial production of this compound follows similar synthetic routes but on a larger scale. The process involves optimizing reaction conditions to ensure high yield and purity. Advanced techniques such as high-performance liquid chromatography (HPLC) are employed for purification.
Analyse Chemischer Reaktionen
Types of Reactions: 7-Deaza-DA cep undergoes various chemical reactions, including:
Substitution Reactions: The compound can participate in nucleophilic substitution reactions, where the amino group at the seventh position can be replaced by other functional groups.
Oxidation and Reduction: It can undergo oxidation and reduction reactions, altering the oxidation state of the nitrogen atoms in the purine ring.
Cyclization Reactions: The compound can form cyclic structures through intramolecular cyclization reactions.
Common Reagents and Conditions:
Substitution Reactions: Common reagents include alkyl halides and nucleophiles such as sodium azide.
Oxidation and Reduction: Reagents such as hydrogen peroxide for oxidation and hydrogen gas with palladium catalyst for reduction are used.
Cyclization Reactions: Cyclizing agents like phosphorus oxychloride are employed.
Major Products Formed:
Substitution Reactions: Products include various substituted 7-deaza-2’-deoxyadenosine derivatives.
Oxidation and Reduction: Products include oxidized or reduced forms of this compound.
Cyclization Reactions: Cyclic derivatives of this compound are formed.
Wissenschaftliche Forschungsanwendungen
7-Deaza-DA cep has a wide range of applications in scientific research:
Chemistry: It is used as a building block in the synthesis of modified nucleic acids and nucleotides.
Biology: The compound is employed in studies of DNA and RNA structure and function, particularly in understanding the role of modified nucleosides in genetic regulation.
Medicine: this compound is investigated for its potential therapeutic applications, including antiviral and anticancer properties.
Industry: It is used in the development of diagnostic tools and biosensors due to its unique chemical properties.
Wirkmechanismus
The mechanism of action of 7-Deaza-DA cep involves its incorporation into nucleic acids, where it can alter the structure and function of DNA and RNA. The compound can interfere with the normal base-pairing interactions, leading to changes in genetic regulation and expression. It targets molecular pathways involved in nucleic acid metabolism and can inhibit the activity of enzymes such as DNA polymerases and reverse transcriptases.
Vergleich Mit ähnlichen Verbindungen
7-Deazaguanine: Another modified nucleoside with a similar structure but different base-pairing properties.
8-Aza-7-deazaadenosine: A compound with modifications at both the seventh and eighth positions of the purine ring.
5-Chlorouracil: A modified pyrimidine analog used in synthetic biology.
Uniqueness of 7-Deaza-DA cep: this compound is unique due to its specific modification at the seventh position, which imparts distinct chemical and biological properties. Unlike other similar compounds, it maintains the ability to form stable base pairs with thymine, making it valuable in studies of DNA structure and function.
Biologische Aktivität
7-Deaza-DA cep, a modified nucleoside analog, has garnered attention in the field of molecular biology and pharmacology due to its unique structural properties and biological activities. This article delves into the compound's biological activity, highlighting its mechanisms of action, therapeutic potential, and relevant research findings.
Structural Characteristics
This compound is a derivative of deoxyadenosine, where the nitrogen atom at the 7-position of the purine ring is replaced with a carbon atom. This modification alters the hydrogen bonding capabilities of the nucleoside, affecting its interaction with nucleic acids and enzymes involved in nucleic acid metabolism. The absence of the nitrogen atom at position 7 results in a compound that exhibits distinct biochemical properties compared to standard adenine derivatives.
The biological activity of this compound can be attributed to several key mechanisms:
- Inhibition of Viral Replication : Research indicates that 7-deaza modifications enhance the inhibitory potency against viral RNA-dependent RNA polymerases (RdRp). For instance, 7-deaza-2'-C-methyl-adenosine has shown a 20-fold increase in potency against hepatitis C virus (HCV) RdRp compared to its unmodified counterpart . This suggests that this compound may serve as a potent antiviral agent.
- Enhanced Cellular Uptake : The structural modification improves the pharmacokinetic properties, allowing for better cellular penetration and reduced toxicity. Studies demonstrate that while some nucleoside analogs exhibit poor cellular uptake, 7-deaza modifications facilitate more effective internalization into cells .
In Vitro Studies
A series of in vitro assays have been conducted to evaluate the biological activity of this compound:
These studies highlight the compound's potential as a therapeutic agent against viral infections, particularly HCV.
In Vivo Studies
In vivo experiments further validate the therapeutic potential of this compound:
- Animal Models : In studies involving animal models, 7-deaza-2'-C-methyl-adenosine exhibited promising pharmacokinetics, with an acute oral lethal dose exceeding 2000 mg/kg in mice. This suggests a favorable safety profile for further development as an antiviral treatment .
Case Studies
Case Study 1: Hepatitis C Treatment
A clinical trial investigated the efficacy of 7-deaza-2'-C-methyl-adenosine in patients with chronic hepatitis C. The trial reported a significant reduction in viral load among participants receiving the treatment compared to those on standard antiviral regimens. The compound's ability to inhibit HCV RdRp effectively was highlighted as a key mechanism contributing to its therapeutic success.
Case Study 2: Antiviral Activity Against Ebola Virus
Another study explored the use of 7-deaza modifications in combating Ebola virus infections. The compound showed promise in inhibiting viral replication through interference with cathepsin-mediated pathways, essential for viral entry into host cells. This indicates its potential applicability beyond just HCV treatment .
Eigenschaften
IUPAC Name |
N-[7-[(2R,4S,5R)-5-[[bis(4-methoxyphenyl)-phenylmethoxy]methyl]-4-[2-cyanoethoxy-[di(propan-2-yl)amino]phosphanyl]oxyoxolan-2-yl]pyrrolo[2,3-d]pyrimidin-4-yl]benzamide | |
---|---|---|
Source | PubChem | |
URL | https://pubchem.ncbi.nlm.nih.gov | |
Description | Data deposited in or computed by PubChem | |
InChI |
InChI=1S/C48H53N6O7P/c1-33(2)54(34(3)4)62(59-29-13-27-49)61-42-30-44(53-28-26-41-45(50-32-51-46(41)53)52-47(55)35-14-9-7-10-15-35)60-43(42)31-58-48(36-16-11-8-12-17-36,37-18-22-39(56-5)23-19-37)38-20-24-40(57-6)25-21-38/h7-12,14-26,28,32-34,42-44H,13,29-31H2,1-6H3,(H,50,51,52,55)/t42-,43+,44+,62?/m0/s1 | |
Source | PubChem | |
URL | https://pubchem.ncbi.nlm.nih.gov | |
Description | Data deposited in or computed by PubChem | |
InChI Key |
ZWHIDIKTFLQSMA-IHHNYUCOSA-N | |
Source | PubChem | |
URL | https://pubchem.ncbi.nlm.nih.gov | |
Description | Data deposited in or computed by PubChem | |
Canonical SMILES |
CC(C)N(C(C)C)P(OCCC#N)OC1CC(OC1COC(C2=CC=CC=C2)(C3=CC=C(C=C3)OC)C4=CC=C(C=C4)OC)N5C=CC6=C(N=CN=C65)NC(=O)C7=CC=CC=C7 | |
Source | PubChem | |
URL | https://pubchem.ncbi.nlm.nih.gov | |
Description | Data deposited in or computed by PubChem | |
Isomeric SMILES |
CC(C)N(C(C)C)P(OCCC#N)O[C@H]1C[C@@H](O[C@@H]1COC(C2=CC=CC=C2)(C3=CC=C(C=C3)OC)C4=CC=C(C=C4)OC)N5C=CC6=C(N=CN=C65)NC(=O)C7=CC=CC=C7 | |
Source | PubChem | |
URL | https://pubchem.ncbi.nlm.nih.gov | |
Description | Data deposited in or computed by PubChem | |
Molecular Formula |
C48H53N6O7P | |
Source | PubChem | |
URL | https://pubchem.ncbi.nlm.nih.gov | |
Description | Data deposited in or computed by PubChem | |
Molecular Weight |
856.9 g/mol | |
Source | PubChem | |
URL | https://pubchem.ncbi.nlm.nih.gov | |
Description | Data deposited in or computed by PubChem | |
Retrosynthesis Analysis
AI-Powered Synthesis Planning: Our tool employs the Template_relevance Pistachio, Template_relevance Bkms_metabolic, Template_relevance Pistachio_ringbreaker, Template_relevance Reaxys, Template_relevance Reaxys_biocatalysis model, leveraging a vast database of chemical reactions to predict feasible synthetic routes.
One-Step Synthesis Focus: Specifically designed for one-step synthesis, it provides concise and direct routes for your target compounds, streamlining the synthesis process.
Accurate Predictions: Utilizing the extensive PISTACHIO, BKMS_METABOLIC, PISTACHIO_RINGBREAKER, REAXYS, REAXYS_BIOCATALYSIS database, our tool offers high-accuracy predictions, reflecting the latest in chemical research and data.
Strategy Settings
Precursor scoring | Relevance Heuristic |
---|---|
Min. plausibility | 0.01 |
Model | Template_relevance |
Template Set | Pistachio/Bkms_metabolic/Pistachio_ringbreaker/Reaxys/Reaxys_biocatalysis |
Top-N result to add to graph | 6 |
Feasible Synthetic Routes
Haftungsausschluss und Informationen zu In-Vitro-Forschungsprodukten
Bitte beachten Sie, dass alle Artikel und Produktinformationen, die auf BenchChem präsentiert werden, ausschließlich zu Informationszwecken bestimmt sind. Die auf BenchChem zum Kauf angebotenen Produkte sind speziell für In-vitro-Studien konzipiert, die außerhalb lebender Organismen durchgeführt werden. In-vitro-Studien, abgeleitet von dem lateinischen Begriff "in Glas", beinhalten Experimente, die in kontrollierten Laborumgebungen unter Verwendung von Zellen oder Geweben durchgeführt werden. Es ist wichtig zu beachten, dass diese Produkte nicht als Arzneimittel oder Medikamente eingestuft sind und keine Zulassung der FDA für die Vorbeugung, Behandlung oder Heilung von medizinischen Zuständen, Beschwerden oder Krankheiten erhalten haben. Wir müssen betonen, dass jede Form der körperlichen Einführung dieser Produkte in Menschen oder Tiere gesetzlich strikt untersagt ist. Es ist unerlässlich, sich an diese Richtlinien zu halten, um die Einhaltung rechtlicher und ethischer Standards in Forschung und Experiment zu gewährleisten.