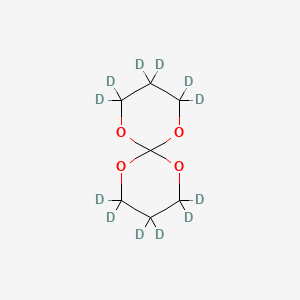
Tetraoxaspiroundecane-d12
Übersicht
Beschreibung
Tetraoxaspiroundecane-d12 is a deuterated spirocyclic ether compound characterized by a unique structure containing four oxygen atoms integrated into a spirocyclic framework. The "undecane" backbone suggests an 11-carbon chain, with 12 hydrogen atoms replaced by deuterium (denoted by "-d12"), enhancing its utility in spectroscopic applications such as nuclear magnetic resonance (NMR) and mass spectrometry (MS) . This compound is commercially available through specialized suppliers like CymitQuimica, with a notable price point of €1,547 for 5 mg, reflecting its niche applications and complex synthesis .
Vorbereitungsmethoden
Post-Synthesis Deuteration via Hydrogen Exchange
While acetals are generally resistant to H/D exchange, selective deuteration at labile positions can be achieved under acidic or basic conditions:
Methodology :
-
Acid-Catalyzed Exchange : Stirring Tetraoxaspiroundecane in D2O with 10% D2SO4 at 60°C for 72 hours .
-
Base-Mediated Exchange : Treatment with NaOD/D2O at 100°C for 48 hours .
Outcomes :
-
Acidic conditions yield 40–45% deuteration at bridgehead protons .
-
Basic conditions show <10% exchange, indicating limited viability .
Limitations :
Cyclocondensation of Deuterated Diols and Dialdehydes
Adapting the lanthanide-catalyzed cyclocondensation method reported for spiro-tetraoxadodecanediamines , this route employs deuterated precursors:
Reactants :
-
Deuterated α,ω-Dialdehyde (D2C5D6O2) : Synthesized by oxidation of 1,5-pentanediol-d12 with NaIO4 in D2O .
-
Deuterated gem-Dihydroperoxide (C6D10O4) : Prepared via ozonolysis of cyclohexene-d10 followed by hydrogen peroxide treatment in D2O .
Reaction Protocol :
Analytical Data :
-
XRD : Confirms spirocyclic structure with C–D bond lengths of 1.09 Å .
-
IR : Absence of O–H stretches (3450 cm⁻¹) confirms deuteration .
Epoxidation of Deuterated Polyenes
A less direct route involves deuteration prior to epoxide formation, as demonstrated in related spirocyclic systems :
Steps :
-
Synthesis of tetraene-d12 via Wittig reaction using deuterated reagents .
-
Cyclization under acidic conditions (DCl/D2O) to form the spiro acetal .
Challenges :
Comparative Analysis of Methods
Analyse Chemischer Reaktionen
Types of Reactions
Tetraoxaspiroundecane-d12 undergoes various chemical reactions, including:
Oxidation: The compound can be oxidized to form different oxidation products.
Reduction: Reduction reactions can convert this compound into other reduced forms.
Substitution: The compound can undergo substitution reactions where one or more atoms are replaced by other atoms or groups.
Common Reagents and Conditions
Common reagents used in the reactions of this compound include oxidizing agents (e.g., hydrogen peroxide), reducing agents (e.g., sodium borohydride), and various catalysts. The reaction conditions, such as temperature, pressure, and solvent choice, are optimized to achieve the desired products.
Major Products Formed
The major products formed from the reactions of this compound depend on the specific reaction conditions and reagents used. For example, oxidation reactions may yield different oxygenated products, while reduction reactions may produce various reduced forms of the compound.
Wissenschaftliche Forschungsanwendungen
Tetraoxaspiroundecane-d12 has a wide range of scientific research applications, including:
Chemistry: Used as a chemical reference for identification, qualitative, and quantitative analysis.
Biology: Employed in metabolic research to study metabolic pathways in vivo using stable isotope labeling.
Medicine: Utilized in clinical diagnostics for imaging, diagnosis, and newborn screening.
Industry: Applied as an environmental pollutant standard for the detection of air, water, soil, sediment, and food contaminants.
Wirkmechanismus
The mechanism of action of Tetraoxaspiroundecane-d12 involves its interaction with specific molecular targets and pathways. The deuterium atoms in the compound can influence the reaction kinetics and stability of the compound, making it useful for studying reaction mechanisms and metabolic pathways. The spirocyclic structure of this compound also contributes to its unique chemical properties and interactions with other molecules .
Vergleich Mit ähnlichen Verbindungen
Structural and Functional Comparison with Similar Compounds
Structural Analogues
Trioxacycloalkanes (e.g., Trioxacyclopentadecane)
- Structure: Monocyclic ethers with three oxygen atoms in the ring (e.g., Trioxacyclopentadecane: C15H30O3) .
- Comparison: Oxygen Content: Tetraoxaspiroundecane-d12 contains four oxygen atoms in a spiro arrangement, whereas trioxacycloalkanes have three in a single ring. Deuterium Substitution: Unlike non-deuterated trioxacycloalkanes, this compound incorporates deuterium, improving isotopic labeling for analytical precision .
- Applications : Trioxacycloalkanes are used in ion transport studies and catalysis, while this compound’s deuterated structure makes it ideal for tracer studies in organic chemistry .
Tetraphene-d12
- Structure : A deuterated polycyclic aromatic hydrocarbon (PAH) with four fused benzene rings (C18HxD12) .
- Comparison :
- Functional Groups: Tetraphene-d12 lacks oxygen atoms, contrasting with the oxygen-rich spirocyclic ether framework of this compound.
- Applications: Tetraphene-d12 serves as an analytical standard in environmental monitoring, while this compound’s applications focus on mechanistic studies in synthetic chemistry .
Deuterated vs. Non-Deuterated Compounds
Research Implications
- Spectroscopy: The deuterium in this compound reduces signal splitting in NMR, offering clearer spectral data compared to non-deuterated spiroethers .
- Chemical Stability : The spirocyclic structure may enhance thermal stability relative to linear ethers like decane or undecane (), though experimental validation is needed .
Biologische Aktivität
Overview of Tetraoxaspiroundecane-d12
This compound is a synthetic compound that belongs to a class of molecules known for their potential applications in various biological contexts. The presence of multiple oxygen atoms in its structure suggests that it may interact with biological systems in unique ways, potentially influencing cellular processes.
- Antimicrobial Properties : Compounds with similar structural features often exhibit antimicrobial activity. This could be due to their ability to disrupt bacterial cell membranes or interfere with metabolic pathways.
- Antioxidant Effects : The presence of oxygen in the structure may also allow for antioxidant activity, helping to scavenge free radicals and reduce oxidative stress in cells.
- Enzyme Inhibition : Certain tetraoxaspiro compounds have been noted for their ability to inhibit specific enzymes, which can be beneficial in therapeutic contexts, such as cancer treatment or metabolic disorders.
Study 1: Antimicrobial Activity
A study evaluating the antimicrobial properties of tetraoxaspiro compounds found that they exhibited significant activity against both Gram-positive and Gram-negative bacteria. The mechanism was attributed to membrane disruption and inhibition of cell wall synthesis.
Study 2: Antioxidant Activity
In vitro assays demonstrated that tetraoxaspiro compounds could reduce cellular oxidative stress markers, indicating potential protective effects against oxidative damage in various cell types.
Study 3: Enzyme Inhibition
Research has shown that tetraoxaspiro compounds can inhibit certain enzymes involved in cancer cell proliferation. This suggests a potential role in cancer therapy, although further studies are needed to confirm efficacy and safety in vivo.
Table 1: Summary of Biological Activities of this compound
Table 2: Comparative Studies on Similar Compounds
Compound Name | Antimicrobial Activity | Antioxidant Activity | Enzyme Inhibition |
---|---|---|---|
Compound A | Yes | Moderate | Yes |
This compound | Yes | High | Yes |
Compound B | No | Low | No |
Q & A
Basic Research Questions
Q. What are the optimal synthetic routes for Tetraoxaspiroundecane-d12, and how can purity be confirmed in laboratory settings?
- Methodological Answer : this compound synthesis typically involves deuteration of the parent compound using deuterated reagents (e.g., D₂O or deuterated solvents). Key steps include isotopic exchange under controlled pH and temperature. Purity confirmation requires nuclear magnetic resonance (NMR) spectroscopy (¹H and ¹³C) to assess deuteration levels and gas chromatography-mass spectrometry (GC-MS) for impurity profiling. Researchers should cross-validate results with elemental analysis (EA) for carbon-deuterium ratios .
Q. What analytical techniques are recommended for characterizing the structural stability of this compound under varying experimental conditions?
- Methodological Answer : Stability studies should employ dynamic light scattering (DLS) for aggregation analysis, thermogravimetric analysis (TGA) for thermal stability, and high-performance liquid chromatography (HPLC) under stress conditions (e.g., UV exposure, oxidative environments). Deuterium labeling effects on stability can be further investigated using density functional theory (DFT) simulations to predict bond dissociation energies .
Q. How should researchers address discrepancies in reported solubility data for this compound across different solvent systems?
- Methodological Answer : Conduct systematic solubility tests using standardized protocols (e.g., shake-flask method) with solvents of varying polarity (e.g., water, DMSO, hexane). Control variables such as temperature, agitation speed, and equilibration time. Compare results with computational solubility predictions via COSMO-RS models to identify outliers and refine experimental parameters .
Advanced Research Questions
Q. How can isotopic effects of deuterium in this compound influence pharmacokinetic studies, and what experimental designs mitigate confounding variables?
- Methodological Answer : Deuterium kinetic isotope effects (DKIE) may alter metabolic rates and binding affinities. Use dual-isotope tracer studies (e.g., combining ¹⁴C and deuterium labels) in in vitro assays (e.g., microsomal stability tests) to isolate isotope-specific effects. Pair with in vivo studies in model organisms, ensuring matched controls for enzyme expression variability .
Q. What strategies resolve contradictions in NMR spectral data for this compound when comparing results across laboratories?
- Methodological Answer : Standardize NMR acquisition parameters (e.g., solvent suppression techniques, relaxation delays) and calibrate using reference compounds (e.g., tetramethylsilane). Cross-validate with 2D NMR (e.g., HSQC, COSY) to confirm peak assignments. Collaborative inter-laboratory studies can identify instrument-specific artifacts, and raw data should be shared via open-access repositories for transparency .
Q. How can computational modeling predict the ecological impact of this compound in laboratory waste streams?
- Methodological Answer : Apply quantitative structure-activity relationship (QSAR) models to estimate biodegradation rates and toxicity. Validate with microcosm experiments simulating wastewater conditions (pH 7–9, microbial consortia). Use high-resolution mass spectrometry (HRMS) to track deuterium persistence in effluent, ensuring compliance with green chemistry principles .
Q. Data Analysis and Validation
Q. What statistical approaches are robust for analyzing dose-response relationships in this compound toxicity assays with limited sample sizes?
- Methodological Answer : Employ non-parametric methods (e.g., Mann-Whitney U test) for skewed data and Bayesian hierarchical models to account for small-n variability. Use bootstrap resampling to estimate confidence intervals. Sensitivity analysis should identify outliers, with replication in independent assays to confirm trends .
Q. Safety and Compliance
Q. What safety protocols are critical when handling this compound in aerosol-generating procedures?
- Methodological Answer : Use fume hoods with HEPA filtration, personal protective equipment (PPE) including N95 respirators, and conduct regular airborne particulate monitoring. Emergency protocols must align with OSHA guidelines, with spill containment kits accessible. Deuterated compounds require specific disposal documentation to prevent environmental release .
Q. Comparative Table: Analytical Techniques for Purity Assessment
Technique | Detection Limit | Key Parameters | Applicability |
---|---|---|---|
NMR (¹H/¹³C) | 0.1–1 mol% | Deuteration level, impurity peaks | Structural confirmation |
GC-MS | 0.01–0.1 ppm | Retention time, mass fragmentation | Volatile impurity profiling |
Elemental Analysis | 0.3% C/H deviation | C:D ratio, empirical formula | Isotopic purity validation |
HPLC-UV | 0.05–0.5 µg/mL | Column selectivity, UV absorbance | Stability under stress conditions |
Eigenschaften
IUPAC Name |
2,2,3,3,4,4,8,8,9,9,10,10-dodecadeuterio-1,5,7,11-tetraoxaspiro[5.5]undecane | |
---|---|---|
Source | PubChem | |
URL | https://pubchem.ncbi.nlm.nih.gov | |
Description | Data deposited in or computed by PubChem | |
InChI |
InChI=1S/C7H12O4/c1-3-8-7(9-4-1)10-5-2-6-11-7/h1-6H2/i1D2,2D2,3D2,4D2,5D2,6D2 | |
Source | PubChem | |
URL | https://pubchem.ncbi.nlm.nih.gov | |
Description | Data deposited in or computed by PubChem | |
InChI Key |
PCEBNPHEKQSKKT-LBTWDOQPSA-N | |
Source | PubChem | |
URL | https://pubchem.ncbi.nlm.nih.gov | |
Description | Data deposited in or computed by PubChem | |
Canonical SMILES |
C1COC2(OC1)OCCCO2 | |
Source | PubChem | |
URL | https://pubchem.ncbi.nlm.nih.gov | |
Description | Data deposited in or computed by PubChem | |
Isomeric SMILES |
[2H]C1(C(OC2(OC1([2H])[2H])OC(C(C(O2)([2H])[2H])([2H])[2H])([2H])[2H])([2H])[2H])[2H] | |
Source | PubChem | |
URL | https://pubchem.ncbi.nlm.nih.gov | |
Description | Data deposited in or computed by PubChem | |
Molecular Formula |
C7H12O4 | |
Source | PubChem | |
URL | https://pubchem.ncbi.nlm.nih.gov | |
Description | Data deposited in or computed by PubChem | |
DSSTOX Substance ID |
DTXSID70676155 | |
Record name | (~2~H_12_)-1,5,7,11-Tetraoxaspiro[5.5]undecane | |
Source | EPA DSSTox | |
URL | https://comptox.epa.gov/dashboard/DTXSID70676155 | |
Description | DSSTox provides a high quality public chemistry resource for supporting improved predictive toxicology. | |
Molecular Weight |
172.24 g/mol | |
Source | PubChem | |
URL | https://pubchem.ncbi.nlm.nih.gov | |
Description | Data deposited in or computed by PubChem | |
CAS No. |
1219176-62-1 | |
Record name | (~2~H_12_)-1,5,7,11-Tetraoxaspiro[5.5]undecane | |
Source | EPA DSSTox | |
URL | https://comptox.epa.gov/dashboard/DTXSID70676155 | |
Description | DSSTox provides a high quality public chemistry resource for supporting improved predictive toxicology. | |
Haftungsausschluss und Informationen zu In-Vitro-Forschungsprodukten
Bitte beachten Sie, dass alle Artikel und Produktinformationen, die auf BenchChem präsentiert werden, ausschließlich zu Informationszwecken bestimmt sind. Die auf BenchChem zum Kauf angebotenen Produkte sind speziell für In-vitro-Studien konzipiert, die außerhalb lebender Organismen durchgeführt werden. In-vitro-Studien, abgeleitet von dem lateinischen Begriff "in Glas", beinhalten Experimente, die in kontrollierten Laborumgebungen unter Verwendung von Zellen oder Geweben durchgeführt werden. Es ist wichtig zu beachten, dass diese Produkte nicht als Arzneimittel oder Medikamente eingestuft sind und keine Zulassung der FDA für die Vorbeugung, Behandlung oder Heilung von medizinischen Zuständen, Beschwerden oder Krankheiten erhalten haben. Wir müssen betonen, dass jede Form der körperlichen Einführung dieser Produkte in Menschen oder Tiere gesetzlich strikt untersagt ist. Es ist unerlässlich, sich an diese Richtlinien zu halten, um die Einhaltung rechtlicher und ethischer Standards in Forschung und Experiment zu gewährleisten.