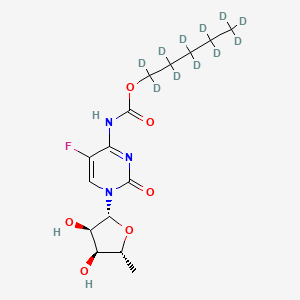
Capecitabine-d11
Beschreibung
Eigenschaften
IUPAC Name |
1,1,2,2,3,3,4,4,5,5,5-undecadeuteriopentyl N-[1-[(2R,3R,4S,5R)-3,4-dihydroxy-5-methyloxolan-2-yl]-5-fluoro-2-oxopyrimidin-4-yl]carbamate | |
---|---|---|
Source | PubChem | |
URL | https://pubchem.ncbi.nlm.nih.gov | |
Description | Data deposited in or computed by PubChem | |
InChI |
InChI=1S/C15H22FN3O6/c1-3-4-5-6-24-15(23)18-12-9(16)7-19(14(22)17-12)13-11(21)10(20)8(2)25-13/h7-8,10-11,13,20-21H,3-6H2,1-2H3,(H,17,18,22,23)/t8-,10-,11-,13-/m1/s1/i1D3,3D2,4D2,5D2,6D2 | |
Source | PubChem | |
URL | https://pubchem.ncbi.nlm.nih.gov | |
Description | Data deposited in or computed by PubChem | |
InChI Key |
GAGWJHPBXLXJQN-XSFNBKHLSA-N | |
Source | PubChem | |
URL | https://pubchem.ncbi.nlm.nih.gov | |
Description | Data deposited in or computed by PubChem | |
Canonical SMILES |
CCCCCOC(=O)NC1=NC(=O)N(C=C1F)C2C(C(C(O2)C)O)O | |
Source | PubChem | |
URL | https://pubchem.ncbi.nlm.nih.gov | |
Description | Data deposited in or computed by PubChem | |
Isomeric SMILES |
[2H]C([2H])([2H])C([2H])([2H])C([2H])([2H])C([2H])([2H])C([2H])([2H])OC(=O)NC1=NC(=O)N(C=C1F)[C@H]2[C@@H]([C@@H]([C@H](O2)C)O)O | |
Source | PubChem | |
URL | https://pubchem.ncbi.nlm.nih.gov | |
Description | Data deposited in or computed by PubChem | |
Molecular Formula |
C15H22FN3O6 | |
Source | PubChem | |
URL | https://pubchem.ncbi.nlm.nih.gov | |
Description | Data deposited in or computed by PubChem | |
DSSTOX Substance ID |
DTXSID90678974 | |
Record name | 5'-Deoxy-5-fluoro-N-{[(~2~H_11_)pentyloxy]carbonyl}cytidine | |
Source | EPA DSSTox | |
URL | https://comptox.epa.gov/dashboard/DTXSID90678974 | |
Description | DSSTox provides a high quality public chemistry resource for supporting improved predictive toxicology. | |
Molecular Weight |
370.42 g/mol | |
Source | PubChem | |
URL | https://pubchem.ncbi.nlm.nih.gov | |
Description | Data deposited in or computed by PubChem | |
CAS No. |
1132662-08-8 | |
Record name | 5'-Deoxy-5-fluoro-N-{[(~2~H_11_)pentyloxy]carbonyl}cytidine | |
Source | EPA DSSTox | |
URL | https://comptox.epa.gov/dashboard/DTXSID90678974 | |
Description | DSSTox provides a high quality public chemistry resource for supporting improved predictive toxicology. | |
Wirkmechanismus
Target of Action
Capecitabine-d11, like its parent compound Capecitabine, is a prodrug that is enzymatically converted to fluorouracil (an antimetabolite) in the tumor. The primary targets of this compound are the cancerous cells where it interferes with DNA synthesis.
Mode of Action
Once inside the cancerous cells, this compound is converted to fluorouracil. Fluorouracil is a fluorinated pyrimidine antimetabolite that inhibits thymidylate synthetase, blocking the methylation of deoxyuridylic acid to thymidylic acid, interfering with DNA, and to a lesser degree, RNA synthesis. This interaction with its targets leads to the slowing of tumor tissue growth.
Biochemical Pathways
The biochemical pathways affected by this compound involve the conversion of the prodrug to its active form, fluorouracil, and the subsequent inhibition of DNA synthesis. The conversion process involves several steps, including the action of enzymes such as carboxylesterase and cytidine deaminase. The inhibition of DNA synthesis disrupts the normal cell cycle, particularly the G1 and S phases.
Pharmacokinetics
The pharmacokinetics of this compound involves its absorption, distribution, metabolism, and excretion (ADME). After oral administration, this compound is rapidly and extensively absorbed. It is metabolized in the liver and tissues to form fluorouracil, which is the active moiety. The inactive metabolites are mainly excreted in the urine. The terminal half-life of this compound is approximately 0.75 hours.
Result of Action
The molecular and cellular effects of this compound’s action involve the inhibition of DNA synthesis and the slowing of tumor tissue growth. This results in the death of cancerous cells, thereby reducing the size of the tumor and slowing the progression of the disease.
Action Environment
The action, efficacy, and stability of this compound can be influenced by various environmental factors. For instance, the presence of certain enzymes in the tumor environment is crucial for the conversion of this compound to its active form. Additionally, factors such as the patient’s renal function can impact the drug’s excretion and overall effectiveness.
Biochemische Analyse
Biochemical Properties
Capecitabine-d11, like its parent compound Capecitabine, interacts with various enzymes and proteins in the body. It is metabolized in three steps to form 5-fluorouracil. The first step is hydrolysis by carboxylesterase to form 5’-deoxy-5-fluorocytidine (5’-DFCR). This is then converted to 5’-deoxy-5-fluorouridine (5’-DFUR) by cytidine deaminase. Finally, thymidine phosphorylase converts 5’-DFUR to the active drug, 5-fluorouracil. These interactions are crucial for the activation of this compound and its subsequent therapeutic effect.
Cellular Effects
This compound affects various types of cells and cellular processes, primarily cancer cells. The active metabolite, 5-fluorouracil, inhibits DNA synthesis and slows the growth of tumor tissue. It impacts cell signaling pathways, gene expression, and cellular metabolism, leading to the death of cancer cells
Molecular Mechanism
The molecular mechanism of action of this compound involves its conversion to 5-fluorouracil, which exerts its effects at the molecular level. 5-fluorouracil inhibits the enzyme thymidylate synthase, disrupting DNA synthesis and leading to cell death. It also incorporates into RNA and DNA, causing further disruption of essential cellular processes.
Temporal Effects in Laboratory Settings
Studies on Capecitabine have shown that it is well-tolerated over extended periods.
Dosage Effects in Animal Models
The effects of this compound at different dosages in animal models have not been extensively studied. Studies on Capecitabine have shown that its effects can vary with dosage, with higher doses potentially leading to increased toxicity.
Metabolic Pathways
This compound is involved in several metabolic pathways. After oral administration, it is metabolized in the liver and tumor tissues to form 5-fluorouracil. This process involves the enzymes carboxylesterase, cytidine deaminase, and thymidine phosphorylase.
Biologische Aktivität
Chemical Structure and Properties
Capecitabine-d11 is characterized by the replacement of 11 hydrogen atoms with deuterium isotopes in the capecitabine molecule. This modification results in a slightly higher molecular weight but does not significantly alter its chemical properties or behavior during analysis. The molecular formula for this compound is with a molecular weight of approximately 370.418 g/mol.
Capecitabine itself is an oral prodrug that is metabolized to 5-fluorouracil (5-FU), an active chemotherapeutic agent, through a series of enzymatic reactions. The conversion occurs primarily in tumor tissues where thymidine phosphorylase catalyzes the final step, leading to localized drug activation. However, this compound does not exhibit distinct biological activity; rather, it functions as an internal standard to improve the quantification of capecitabine and its metabolites.
Absorption and Metabolism
Capecitabine is rapidly absorbed from the gastrointestinal tract and undergoes extensive metabolism. The pharmacokinetic profile includes:
- Peak Plasma Concentrations (Cmax) : Achieved approximately 1.5 to 3.5 hours post-administration.
- Elimination Half-Life (t1/2) : Ranges from 1 to 3 hours for capecitabine and its metabolites.
- Metabolites : Key metabolites include 5′-deoxy-5-fluorocytidine (5′-DFCR), 5′-deoxy-5-fluorouridine (5′-DFUR), and 5-FU.
Variability
Pharmacokinetic variability can be influenced by factors such as food intake, renal function, liver function, and genetic polymorphisms affecting drug metabolism enzymes. For instance, patients with liver dysfunction may exhibit altered plasma concentrations of capecitabine and its metabolites.
Therapeutic Drug Monitoring
This compound's primary application lies in therapeutic drug monitoring (TDM) for capecitabine treatment regimens. By serving as a stable isotopic internal standard, it helps minimize errors during LC-MS/MS analysis, thus ensuring reliable quantification of drug levels in biological matrices like plasma or dried blood spots.
Case Studies
Several studies have highlighted the importance of monitoring capecitabine levels for optimizing treatment outcomes:
- Study on Canines : A pilot study involving dogs with carcinomas demonstrated that capecitabine was well-tolerated at a dosage of 750 mg/m². The median area under the concentration-time curve (AUC) was reported at 890 hng/ml, indicating effective absorption and metabolism similar to that observed in humans.
- Human Pharmacokinetics : Research has shown that adherence to dosing regimens significantly impacts plasma concentrations of capecitabine and its metabolites, which correlate with treatment efficacy and toxicity outcomes.
Q & A
Basic Research Questions
Q. What is the scientific rationale for using deuterated analogs like Capecitabine-d11 in pharmacokinetic studies?
Deuterated compounds such as this compound are used to track drug metabolism and distribution due to the isotopic mass difference, which allows precise detection via mass spectrometry. Deuterium substitution at specific positions (e.g., 11 hydrogen atoms replaced) reduces metabolic degradation rates, extending the half-life and improving traceability in biological matrices. This approach is critical for distinguishing endogenous molecules from administered drugs in complex systems .
Q. What are the key analytical techniques for characterizing this compound purity and isotopic enrichment?
Researchers should employ:
- Nuclear Magnetic Resonance (NMR) : To confirm deuterium substitution patterns and structural integrity.
- High-Performance Liquid Chromatography (HPLC) with UV detection: To assess chemical purity.
- Mass Spectrometry (MS) : To quantify deuterium enrichment and detect isotopic impurities.
- Stable Isotope Ratio Analysis (SIRA) : For precise measurement of deuterium abundance .
Q. How should researchers design experiments to assess the stability and bioequivalence of this compound compared to non-deuterated Capecitabine?
A robust experimental framework includes:
- Literature Review : Identify metabolic pathways and degradation hotspots of non-deuterated Capecitabine.
- In Vitro Models : Use hepatic microsomes or cell lines to compare metabolic stability.
- In Vivo Pharmacokinetic (PK) Studies : Measure plasma concentration-time profiles in animal models.
- Statistical Methods : Apply ANOVA or mixed-effects models to analyze bioequivalence margins (e.g., 90% CI for AUC and Cmax) .
Advanced Research Questions
Q. How can deuterated this compound be integrated into combination therapy studies to evaluate drug-drug interactions (DDIs)?
- Step 1 : Map overlapping metabolic enzymes (e.g., CYP2C9, CES2) between Capecitabine and co-administered drugs using enzyme inhibition/induction assays.
- Step 2 : Design a crossover PK study in preclinical models, comparing this compound exposure with/without the interacting drug.
- Step 3 : Use physiologically based pharmacokinetic (PBPK) modeling to extrapolate human DDIs, incorporating deuterium-specific metabolic parameters .
Q. What methodological approaches resolve contradictions in studies reporting variable efficacy of deuterated drugs in oncology models?
Contradictions may arise from differences in tumor microenvironments or deuterium placement. Researchers should:
- Conduct Comparative Metabolic Assays : Compare deuterated vs. non-deuterated drug activation in tumor vs. normal tissues.
- Leverage Isotope Tracing : Track deuterium retention in active metabolites (e.g., 5-FU) using LC-MS/MS.
- Validate In Vivo Efficacy : Use orthotopic or patient-derived xenograft (PDX) models with RECIST criteria for tumor response .
Q. How can researchers validate the sensitivity of this compound detection methods in complex biological matrices?
- Matrix-Specific Calibration : Spike known concentrations of this compound into plasma, tumor homogenates, and cerebrospinal fluid.
- Limit of Detection (LOD)/Limit of Quantification (LOQ) : Determine via signal-to-noise ratios (S/N ≥ 3 for LOD; S/N ≥ 10 for LOQ).
- Cross-Validation : Compare results across multiple platforms (e.g., LC-MS vs. MALDI-TOF) .
Q. What experimental designs are optimal for longitudinal studies on this compound metabolic stability in heterogeneous tumor populations?
- Multi-Omics Integration : Pair PK data with transcriptomic profiling of tumor biopsies to identify enzyme expression correlates.
- Serial Sampling : Collect plasma/tumor samples at multiple timepoints to model intra-tumoral drug activation kinetics.
- Cluster Analysis : Stratify patients based on metabolic conversion rates and clinical outcomes .
Q. How do RECIST guidelines apply to preclinical studies evaluating tumor response to deuterated chemotherapeutics?
- Target Lesion Selection : Use imaging (e.g., MRI or PET-CT) to identify measurable lesions.
- Response Criteria : Define complete response (CR), partial response (PR), and progressive disease (PD) based on tumor volume changes.
- Statistical Power : Calculate sample sizes using historical control data from non-deuterated drug trials to ensure detectability of deuterium-specific effects .
Vorbereitungsmethoden
Structural and Molecular Characteristics
Capecitabine-d11 (C₁₅H₁₃D₁₁FN₃O₆, MW 372.43) features 11 deuterium atoms replacing protium at specific positions, enhancing its utility in pharmacokinetic studies. Key properties include:
Property | Value |
---|---|
CAS Number | 1132662-08-8 |
Molecular Formula | C₁₅H₁₃D₁₁FN₃O₆ |
Purity Specifications | ≥99% (by HPLC) |
Storage Conditions | -20°C in airtight containers |
Deuteration typically occurs during synthesis using deuterated reagents or isotopic exchange, though exact positions depend on the synthetic route.
Synthetic Strategy
While detailed protocols for this compound are proprietary, its preparation parallels standard capecitabine synthesis with deuterium incorporation. Key steps derived from non-deuterated routes include:
Step 1: Precursor Synthesis
-
Starting Material : 5'-Deoxy-5-fluorocytidine undergoes protection of hydroxyl groups using trichloromethyl carbonate in dichloromethane/toluene (1:1).
-
Deuterium Introduction : Deuterated solvents (e.g., D₂O) or reagents replace protiated analogs during lactonization or acetylation steps to achieve isotopic labeling.
Step 2: Coupling and Deprotection
Step 3: Purification
Analytical Validation
This compound is quantified using LC-MS/MS with the following parameters:
Parameter | Details |
---|---|
Column | C18 reverse-phase (e.g., Waters Acquity UPLC BEH) |
Mobile Phase | Acetonitrile/0.1% formic acid in water (gradient elution) |
Internal Standard | This compound (3,750 ng/mL in plasma) |
Detection | Positive ion mode (MRM transitions: m/z 359.1 → 179.1 for this compound) |
Linearity Range | 25–2,500 ng/mL (R² > 0.99) |
Applications in Research
Quality Control Metrics
Critical quality attributes for this compound include:
Parameter | Acceptance Criteria |
---|---|
Purity (HPLC) | ≥99% |
Residual Solvents | <0.1% (ICH Q3C guidelines) |
Isotopic Enrichment | ≥98% D-labeling (confirmed by HRMS) |
Featured Recommendations
Most viewed | ||
---|---|---|
Most popular with customers |
Haftungsausschluss und Informationen zu In-Vitro-Forschungsprodukten
Bitte beachten Sie, dass alle Artikel und Produktinformationen, die auf BenchChem präsentiert werden, ausschließlich zu Informationszwecken bestimmt sind. Die auf BenchChem zum Kauf angebotenen Produkte sind speziell für In-vitro-Studien konzipiert, die außerhalb lebender Organismen durchgeführt werden. In-vitro-Studien, abgeleitet von dem lateinischen Begriff "in Glas", beinhalten Experimente, die in kontrollierten Laborumgebungen unter Verwendung von Zellen oder Geweben durchgeführt werden. Es ist wichtig zu beachten, dass diese Produkte nicht als Arzneimittel oder Medikamente eingestuft sind und keine Zulassung der FDA für die Vorbeugung, Behandlung oder Heilung von medizinischen Zuständen, Beschwerden oder Krankheiten erhalten haben. Wir müssen betonen, dass jede Form der körperlichen Einführung dieser Produkte in Menschen oder Tiere gesetzlich strikt untersagt ist. Es ist unerlässlich, sich an diese Richtlinien zu halten, um die Einhaltung rechtlicher und ethischer Standards in Forschung und Experiment zu gewährleisten.