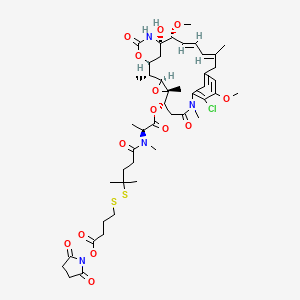
Spdb-DM4
- Klicken Sie auf QUICK INQUIRY, um ein Angebot von unserem Expertenteam zu erhalten.
- Mit qualitativ hochwertigen Produkten zu einem WETTBEWERBSFÄHIGEN Preis können Sie sich mehr auf Ihre Forschung konzentrieren.
Übersicht
Beschreibung
SPDB-DM4 ist eine Verbindung, die bei der Entwicklung von Antikörper-Wirkstoff-Konjugaten (ADCs) verwendet wird. Es ist ein Konjugat des Maytansin-basierten Wirkstoffs DM4, einem potenten Tubulin-Inhibitor, der über einen SPDB-Linker verknüpft ist. Diese Verbindung zeigt eine signifikante Antitumoraktivität und wird hauptsächlich in der Krebsforschung und -behandlung eingesetzt .
Vorbereitungsmethoden
Syntheserouten und Reaktionsbedingungen
Die Herstellung von SPDB-DM4 beinhaltet die Konjugation von DM4 an einen Antikörper über den SPDB-Linker. Der Prozess umfasst in der Regel die folgenden Schritte:
Aktivierung des Antikörpers: Der Antikörper wird mit einem geeigneten Reagenz wie N-Hydroxysuccinimid (NHS)-Ester aktiviert, um reaktive Gruppen einzuführen.
Konjugation: Der aktivierte Antikörper wird dann mit DM4 umgesetzt, das über den SPDB-Linker verknüpft ist.
Industrielle Produktionsverfahren
Die industrielle Produktion von this compound folgt ähnlichen Schritten, jedoch in größerem Maßstab. Der Prozess umfasst:
Aktivierung im Großmaßstab: Aktivierung des Antikörpers in großen Bioreaktoren.
Konjugation und Reinigung: Die Konjugationsreaktion wird im größeren Maßstab durchgeführt, und das resultierende Produkt wird unter Verwendung von Techniken wie Chromatographie gereinigt, um eine hohe Reinheit und Ausbeute zu gewährleisten.
Analyse Chemischer Reaktionen
Arten von Reaktionen
SPDB-DM4 unterliegt verschiedenen Arten von chemischen Reaktionen, darunter:
Reduktion: Die Disulfidbindung im SPDB-Linker kann unter bestimmten Bedingungen reduziert werden.
Substitution: Der Linker kann Substitutionsreaktionen eingehen, bei denen die Disulfidbindung durch andere funktionelle Gruppen ersetzt wird.
Häufige Reagenzien und Bedingungen
Häufig verwendete Reagenzien bei diesen Reaktionen sind Reduktionsmittel wie Dithiothreitol (DTT) und Substitutionsreagenzien wie Thiol-haltige Verbindungen. Diese Reaktionen werden in der Regel unter milden Bedingungen durchgeführt, um die Integrität des Antikörpers zu erhalten .
Hauptprodukte
Die Hauptprodukte, die bei diesen Reaktionen gebildet werden, sind die reduzierte Form von this compound und substituierte Derivate, abhängig von den verwendeten Reagenzien und Bedingungen .
Wissenschaftliche Forschungsanwendungen
SPDB-DM4 hat eine breite Palette von Anwendungen in der wissenschaftlichen Forschung, darunter:
Krebsforschung: Es wird bei der Entwicklung von ADCs für die gezielte Krebstherapie eingesetzt.
Biologische Studien: This compound wird zur Untersuchung der Wirkmechanismen von ADCs und ihrer Interaktionen mit zellulären Zielstrukturen eingesetzt.
Pharmazeutische Entwicklung: Die Verbindung wird bei der Entwicklung neuer Therapeutika und Wirkstoff-Abgabesysteme eingesetzt.
Wirkmechanismus
This compound entfaltet seine Wirkung durch den folgenden Mechanismus:
Targeting von Tumorzellen: Die Antikörperkomponente des ADC zielt auf spezifische Antigene auf der Oberfläche von Tumorzellen ab.
Internalisierung und Freisetzung: Nach der Bindung wird der ADC von der Tumorzelle internalisiert, und der Disulfidlinker wird gespalten, wodurch DM4 freigesetzt wird.
Inhibition der Tubulin-Polymerisation: DM4 bindet an Tubulin und hemmt dessen Polymerisation und stört die Mikrotubuli-Assemblierung. .
Wirkmechanismus
SPDB-DM4 exerts its effects through the following mechanism:
Targeting Tumor Cells: The antibody component of the ADC targets specific antigens on the surface of tumor cells.
Internalization and Release: Upon binding, the ADC is internalized by the tumor cell, and the disulfide linker is cleaved, releasing DM4.
Inhibition of Tubulin Polymerization: DM4 binds to tubulin, inhibiting its polymerization and disrupting microtubule assembly. .
Vergleich Mit ähnlichen Verbindungen
SPDB-DM4 ist einzigartig in seiner Kombination aus SPDB-Linker und DM4-Wirkstoff. Ähnliche Verbindungen umfassen:
MCC-DM1: Eine weitere ADC-Linker-Wirkstoff-Kombination, bei der DM1 über einen Maleimidocaproyl (MCC)-Linker verknüpft ist.
Sulfo-SPDB-DM4: Eine Variante von this compound mit einem sulfonierten SPDB-Linker, der die Löslichkeit erhöht und die Aggregation reduziert.
Diese Verbindungen teilen ähnliche Wirkmechanismen, unterscheiden sich aber in ihrer Linkerchemie und pharmakokinetischen Eigenschaften, was die Einzigartigkeit von this compound in Bezug auf Stabilität und Wirksamkeit unterstreicht .
Eigenschaften
IUPAC Name |
(2,5-dioxopyrrolidin-1-yl) 4-[[5-[[(2S)-1-[[(1S,2R,3S,5S,6S,16E,18E,20R,21S)-11-chloro-21-hydroxy-12,20-dimethoxy-2,5,9,16-tetramethyl-8,23-dioxo-4,24-dioxa-9,22-diazatetracyclo[19.3.1.110,14.03,5]hexacosa-10,12,14(26),16,18-pentaen-6-yl]oxy]-1-oxopropan-2-yl]-methylamino]-2-methyl-5-oxopentan-2-yl]disulfanyl]butanoate |
Source
|
---|---|---|
Source | PubChem | |
URL | https://pubchem.ncbi.nlm.nih.gov | |
Description | Data deposited in or computed by PubChem | |
InChI |
InChI=1S/C46H63ClN4O14S2/c1-26-13-11-14-33(61-10)46(59)25-32(62-43(58)48-46)27(2)41-45(6,64-41)34(24-38(55)50(8)30-22-29(21-26)23-31(60-9)40(30)47)63-42(57)28(3)49(7)35(52)18-19-44(4,5)67-66-20-12-15-39(56)65-51-36(53)16-17-37(51)54/h11,13-14,22-23,27-28,32-34,41,59H,12,15-21,24-25H2,1-10H3,(H,48,58)/b14-11+,26-13+/t27-,28+,32+,33-,34+,41+,45+,46+/m1/s1 |
Source
|
Source | PubChem | |
URL | https://pubchem.ncbi.nlm.nih.gov | |
Description | Data deposited in or computed by PubChem | |
InChI Key |
LAVNQRWLLQGEIB-BAQPUJNESA-N |
Source
|
Source | PubChem | |
URL | https://pubchem.ncbi.nlm.nih.gov | |
Description | Data deposited in or computed by PubChem | |
Canonical SMILES |
CC1C2CC(C(C=CC=C(CC3=CC(=C(C(=C3)OC)Cl)N(C(=O)CC(C4(C1O4)C)OC(=O)C(C)N(C)C(=O)CCC(C)(C)SSCCCC(=O)ON5C(=O)CCC5=O)C)C)OC)(NC(=O)O2)O |
Source
|
Source | PubChem | |
URL | https://pubchem.ncbi.nlm.nih.gov | |
Description | Data deposited in or computed by PubChem | |
Isomeric SMILES |
C[C@@H]1[C@@H]2C[C@]([C@@H](/C=C/C=C(/CC3=CC(=C(C(=C3)OC)Cl)N(C(=O)C[C@@H]([C@]4([C@H]1O4)C)OC(=O)[C@H](C)N(C)C(=O)CCC(C)(C)SSCCCC(=O)ON5C(=O)CCC5=O)C)\C)OC)(NC(=O)O2)O |
Source
|
Source | PubChem | |
URL | https://pubchem.ncbi.nlm.nih.gov | |
Description | Data deposited in or computed by PubChem | |
Molecular Formula |
C46H63ClN4O14S2 |
Source
|
Source | PubChem | |
URL | https://pubchem.ncbi.nlm.nih.gov | |
Description | Data deposited in or computed by PubChem | |
Molecular Weight |
995.6 g/mol |
Source
|
Source | PubChem | |
URL | https://pubchem.ncbi.nlm.nih.gov | |
Description | Data deposited in or computed by PubChem | |
Haftungsausschluss und Informationen zu In-Vitro-Forschungsprodukten
Bitte beachten Sie, dass alle Artikel und Produktinformationen, die auf BenchChem präsentiert werden, ausschließlich zu Informationszwecken bestimmt sind. Die auf BenchChem zum Kauf angebotenen Produkte sind speziell für In-vitro-Studien konzipiert, die außerhalb lebender Organismen durchgeführt werden. In-vitro-Studien, abgeleitet von dem lateinischen Begriff "in Glas", beinhalten Experimente, die in kontrollierten Laborumgebungen unter Verwendung von Zellen oder Geweben durchgeführt werden. Es ist wichtig zu beachten, dass diese Produkte nicht als Arzneimittel oder Medikamente eingestuft sind und keine Zulassung der FDA für die Vorbeugung, Behandlung oder Heilung von medizinischen Zuständen, Beschwerden oder Krankheiten erhalten haben. Wir müssen betonen, dass jede Form der körperlichen Einführung dieser Produkte in Menschen oder Tiere gesetzlich strikt untersagt ist. Es ist unerlässlich, sich an diese Richtlinien zu halten, um die Einhaltung rechtlicher und ethischer Standards in Forschung und Experiment zu gewährleisten.