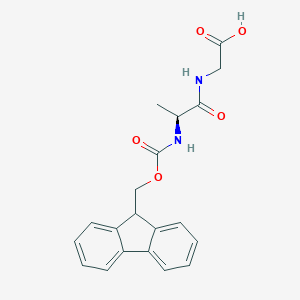
Fmoc-Ala-Gly-OH
Übersicht
Beschreibung
“Fmoc-Ala-Gly-OH” is a compound with the molecular formula C20H20N2O5 . It is also known by other names such as N-(fluorenylmethoxycarbonyl)-L-alanyl-glycine . It is used in peptide synthesis .
Synthesis Analysis
The synthesis of “Fmoc-Ala-Gly-OH” involves the use of FMOC-Ala-OH as a raw material . The synthesis process is optimized to avoid racemization . Standard coupling methods like PyBOP®/DIPEA or DIPCDI/HOBt can be used for their introduction .Molecular Structure Analysis
The molecular weight of “Fmoc-Ala-Gly-OH” is 368.38 g/mol . The IUPAC name is 2-[[ (2S)-2-(9H-fluoren-9-ylmethoxycarbonylamino)propanoyl]amino]acetic acid . The InChIKey is GOCNEQGFDAXBQE-LBPRGKRZSA-N .Chemical Reactions Analysis
“Fmoc-Ala-Gly-OH” is used in Fmoc SPPS for peptide sequences containing Gly . The Fmoc group is rapidly removed by base .Physical And Chemical Properties Analysis
“Fmoc-Ala-Gly-OH” has a predicted boiling point of 678.2±45.0 °C and a predicted density of 1.307±0.06 g/cm3 . It has a pKa value of 3.39±0.10 (Predicted) .Wissenschaftliche Forschungsanwendungen
Biomedical Hydrogels
Fmoc-Ala-Gly-OH: is utilized in the creation of peptide-based hydrogels (PHGs) , which are biocompatible materials suitable for various biomedical applications. These hydrogels can be used for drug delivery and as diagnostic tools for imaging . The self-assembling nature of these peptides allows them to form hydrogels that can support cell adhesion, survival, and duplication, making them potential materials for tissue engineering .
Surface Modification of Nanomaterials
This peptide is also applied in the surface modification of core-shell nanomaterials . For instance, Fmoc-Ala-Gly-OH has been used to modify TiO2@SiO2 core-shell structures to determine the amount of active amino groups on the surface. This is crucial for the development of biosensors and other nanotechnology-based diagnostic tools .
Synthesis of Triazolopeptides
Fmoc-Ala-OH: , a related compound, is used as a building block in the preparation of triazolopeptides . These peptides have applications in the study of protein-protein interactions , enzyme inhibition , and as potential therapeutic agents .
Construction of Antibody-Drug Conjugates
The peptide Fmoc-Gly-Gly-OH , which shares a similar structure, is used as a lysosomally cleavable linker in the construction of antibody-drug conjugates (ADCs) . These ADCs are significant in targeted cancer therapies , where the drug is delivered specifically to cancer cells .
Synthesis of Azapeptides
Fmoc-Ala-OH: is also employed in the synthesis of azapeptides . Azapeptides are analogs of peptides where a nitrogen atom replaces the carbon atom in the backbone. They are studied for their stability against enzymatic degradation and their potential use as peptidomimetics in drug design .
Development of Bis-Cationic Porphyrin Peptides
Another application involves using Fmoc-Ala-OH in the synthesis of bis-cationic porphyrin peptides . These peptides can be used in photodynamic therapy (PDT) for treating cancers. The porphyrin component allows for the generation of reactive oxygen species under light, which can kill cancer cells .
Peptide Materials for Bioprinting
The Fmoc-derivatives of peptides, including Fmoc-Ala-Gly-OH , are proposed as scaffolds for bioprinting applications . Bioprinting is an emerging field that uses 3D printing technology to create complex tissues and organs for regenerative medicine .
Analytical Chemistry Applications
In analytical chemistry, Fmoc-Ala-Gly-OH is used for the quantitative determination of amino groups present on various surfaces. This is important for the characterization of chemical modifications in materials science and the development of functionalized surfaces for various applications .
Safety and Hazards
Zukünftige Richtungen
Wirkmechanismus
Target of Action
Fmoc-Ala-Gly-OH is primarily used in the field of peptide synthesis . The primary targets of this compound are the amino groups of amino acids that are being linked together to form peptides .
Mode of Action
The Fmoc (9-Fluorenylmethoxycarbonyl) group in Fmoc-Ala-Gly-OH acts as a protective group for the amino group during peptide synthesis . It can be introduced by reacting the amine with fluorenylmethyloxycarbonyl chloride (Fmoc-Cl) . The Fmoc group is base-labile, meaning it can be removed by a base . This allows for the sequential addition of amino acids in peptide synthesis .
Biochemical Pathways
Fmoc-Ala-Gly-OH is involved in the biochemical pathway of peptide synthesis . It is used as a building block in the preparation of peptides . The Fmoc group protects the amino group during the coupling reaction, preventing it from reacting with other groups . After the coupling reaction, the Fmoc group is removed, allowing the next amino acid to be added .
Pharmacokinetics
The efficiency of its use in peptide synthesis can be influenced by factors such as the choice of solvent and the specific conditions of the reaction .
Result of Action
The result of the action of Fmoc-Ala-Gly-OH is the formation of peptides with the desired sequence . The Fmoc group allows for the precise addition of amino acids in the correct order . After the peptide has been synthesized, the Fmoc group can be removed, leaving the final peptide product .
Action Environment
The action of Fmoc-Ala-Gly-OH is influenced by the environment in which the peptide synthesis takes place. Factors such as the pH of the solution, the temperature, and the concentration of the reagents can all affect the efficiency of the reaction . The Fmoc group is stable under acidic conditions, but can be removed under basic conditions . This property allows for the selective protection and deprotection of the amino group, which is crucial for the success of peptide synthesis .
Eigenschaften
IUPAC Name |
2-[[(2S)-2-(9H-fluoren-9-ylmethoxycarbonylamino)propanoyl]amino]acetic acid | |
---|---|---|
Source | PubChem | |
URL | https://pubchem.ncbi.nlm.nih.gov | |
Description | Data deposited in or computed by PubChem | |
InChI |
InChI=1S/C20H20N2O5/c1-12(19(25)21-10-18(23)24)22-20(26)27-11-17-15-8-4-2-6-13(15)14-7-3-5-9-16(14)17/h2-9,12,17H,10-11H2,1H3,(H,21,25)(H,22,26)(H,23,24)/t12-/m0/s1 | |
Source | PubChem | |
URL | https://pubchem.ncbi.nlm.nih.gov | |
Description | Data deposited in or computed by PubChem | |
InChI Key |
GOCNEQGFDAXBQE-LBPRGKRZSA-N | |
Source | PubChem | |
URL | https://pubchem.ncbi.nlm.nih.gov | |
Description | Data deposited in or computed by PubChem | |
Canonical SMILES |
CC(C(=O)NCC(=O)O)NC(=O)OCC1C2=CC=CC=C2C3=CC=CC=C13 | |
Source | PubChem | |
URL | https://pubchem.ncbi.nlm.nih.gov | |
Description | Data deposited in or computed by PubChem | |
Isomeric SMILES |
C[C@@H](C(=O)NCC(=O)O)NC(=O)OCC1C2=CC=CC=C2C3=CC=CC=C13 | |
Source | PubChem | |
URL | https://pubchem.ncbi.nlm.nih.gov | |
Description | Data deposited in or computed by PubChem | |
Molecular Formula |
C20H20N2O5 | |
Source | PubChem | |
URL | https://pubchem.ncbi.nlm.nih.gov | |
Description | Data deposited in or computed by PubChem | |
Molecular Weight |
368.4 g/mol | |
Source | PubChem | |
URL | https://pubchem.ncbi.nlm.nih.gov | |
Description | Data deposited in or computed by PubChem | |
Product Name |
Fmoc-Ala-Gly-OH |
Retrosynthesis Analysis
AI-Powered Synthesis Planning: Our tool employs the Template_relevance Pistachio, Template_relevance Bkms_metabolic, Template_relevance Pistachio_ringbreaker, Template_relevance Reaxys, Template_relevance Reaxys_biocatalysis model, leveraging a vast database of chemical reactions to predict feasible synthetic routes.
One-Step Synthesis Focus: Specifically designed for one-step synthesis, it provides concise and direct routes for your target compounds, streamlining the synthesis process.
Accurate Predictions: Utilizing the extensive PISTACHIO, BKMS_METABOLIC, PISTACHIO_RINGBREAKER, REAXYS, REAXYS_BIOCATALYSIS database, our tool offers high-accuracy predictions, reflecting the latest in chemical research and data.
Strategy Settings
Precursor scoring | Relevance Heuristic |
---|---|
Min. plausibility | 0.01 |
Model | Template_relevance |
Template Set | Pistachio/Bkms_metabolic/Pistachio_ringbreaker/Reaxys/Reaxys_biocatalysis |
Top-N result to add to graph | 6 |
Feasible Synthetic Routes
Haftungsausschluss und Informationen zu In-Vitro-Forschungsprodukten
Bitte beachten Sie, dass alle Artikel und Produktinformationen, die auf BenchChem präsentiert werden, ausschließlich zu Informationszwecken bestimmt sind. Die auf BenchChem zum Kauf angebotenen Produkte sind speziell für In-vitro-Studien konzipiert, die außerhalb lebender Organismen durchgeführt werden. In-vitro-Studien, abgeleitet von dem lateinischen Begriff "in Glas", beinhalten Experimente, die in kontrollierten Laborumgebungen unter Verwendung von Zellen oder Geweben durchgeführt werden. Es ist wichtig zu beachten, dass diese Produkte nicht als Arzneimittel oder Medikamente eingestuft sind und keine Zulassung der FDA für die Vorbeugung, Behandlung oder Heilung von medizinischen Zuständen, Beschwerden oder Krankheiten erhalten haben. Wir müssen betonen, dass jede Form der körperlichen Einführung dieser Produkte in Menschen oder Tiere gesetzlich strikt untersagt ist. Es ist unerlässlich, sich an diese Richtlinien zu halten, um die Einhaltung rechtlicher und ethischer Standards in Forschung und Experiment zu gewährleisten.